Abstract
Infertility is a global disorder is resulted from factors related to men or women and affects not only the individual, but also the family and society. Recently, stem cell-based therapy, known as regenerative medicine, uses stem cells or their derivatives to treat diseases, promising new ways to treat infertility. Several in vitro studies as well as studies on animal models, confirm the role of Mesenchymal stem cells (MSC), a kind of multipotent stem cells, in the recovery of folliculogenesis and spermatogenesis. MSCs play the role of therapeutic effect in infertility from two basic aspects including regenerative medicin through differentiation and paracrine pathway via participating in cell homing, immune regulation, and the secretion of active factors and exosomes. Furthermore, there are fewer ethical concerns about using MSCs compared to other source of stem cells like embryonic stem cells. Here we discuss therapeutic approaches of different sources of MSC to restore fertility, then the basic aspects related to their paracrine effects will be described in detail. Finally, methods of MSCs delivery in clinical trials for treatment of infertility-related disorders will be mentioned. But before all these, first we will talk about pros and cons of MSC therapy.
Introduction
Infertility is a social health problem affecting both men and women and is defined as the inability to become pregnant after at least one year of unprotected sex1. Various factors affect couples’ fertility, including genetic factors, anatomical defects, and environmental elements2.
Some major reproductive disorders causing female infertility include uterine abnormalities, ovulatory disorders, a history of tubal pregnancy, and abnormal menstruation3. According to WHO, uterine abnormalities include intrauterine adhesion (IUA; Asherman syndrome [AS]), endometriosis, uterine polyps, and uterine fibroids4. Ovulatory disorders include premature ovarian insufficiency (POI) and polycystic ovary syndrome (PCOS)5.
Similarly, since several factors cause male infertility, problems in males are responsible for almost half of all infertility cases. These factors include hypogonadism (causing low blood testosterone levels), undescended testicles, injured testicles, testicular cancer, varicocele, premature ejaculation, and azoospermia6, 7.
Some common genetic factors also cause infertility, including Turner syndrome, Klein–Felter syndrome, deletion of the azoospermia factor c region of the Y chromosome, fragile X syndrome, androgen receptor (AR) mutations (e.g., CAG repeat expansion), and cystic fibrosis8, 9.
While conventional therapies such as ovulation medications or assisted reproductive technology are effective for treating infertility, they have some limitations10. Therefore, recent studies have focused on evaluating newer methods to treat infertility, such as stem cell transplantation.
Stem cells are self-sustaining cells in an organism that can differentiate into various cell types. Several types of stem cells can be used for different purposes11. Embryonic stem cells (ESCs) are among the most widely used stem cells and can generate differentiated cells with some germ-cell markers. However, their use has disadvantages, such as ethical concerns (destruction of human embryos) and tumor formation12. Therefore, mesenchymal stem cells (MSCs), a new source of stem cells, are an alternative option for infertility treatment. These spindle-shaped cells originate from various tissues and differentiate into cells related to all three germ layers13. Since growth factors secreted by MSCs are involved in survival, proliferation, migration, immunomodulation, and angiogenesis, they have become ideal options for regenerative medicine14. The biological properties of MSCs enable them to regulate immunological mechanisms and contribute to the restoration of the ovaries, ovarian tissue, and uterus. MSCs can also positively affect the treatment of oligozoospermia and azoospermia1.
In this review, we summarize the applications of MSCs in infertility and focus on their potential roles for further research in regenerative medicine.
The pros and cons of MSC therapy
In the past two decades, the emerging fields of stem cell-based therapy have become a new opening for regenerative medicine. Several stem cell types have been found in human tissues, of which the most popular with scientists are MSCs since they have some advantages over other types of stem cell-based therapies for clinical use15. These advantages include their availability, ease of isolation and expansion, broad potential differentiation range, secretion of nutrients for tissue regeneration, dynamic contributions to tissue repair and remodeling after migration to damaged sites, immunoregulatory features, low immunogenicity that allows both autographs and allografts without ethical issues, and limited replicative lifespan16. These unique features provide superiority for MSCs in cellular therapies and make them potential tools in diverse conditions.
While there are currently no clear gold standards or definite markers for identifying MSCs, the International Association of Cell Therapy set the minimum basic criteria for identifying MSCs in 200617: (1) MSCs must have the property of adhering to the culture surface under standard culture conditions; (2) MSCs must express >95% of markers such as 5'-nucleotidase ecto (NT5E/CD73), Thy-1 cell surface antigen (THY1/CD90), and endoglin (ENG/CD105); (3) MSCs must not express >95% of markers such as integrin subunit alpha M (ITGAM/CD11b), CD19, CD34, protein tyrosine phosphatase receptor type C (PTPRC/CD45), CD79a, and human leukocyte antigen–DR isotype (HLA-DR); (4) MSCs must be able to differentiate into adipocytes, chondroblasts, and osteoblasts in vitro18. While these positive markers describe MSCs, no specific marker has been confirmed for MSCs alone19. It should also be noted that MSCs’ proliferation and differentiation capacities may differ markedly between their various sources. These differences appear to be due to the direct impact of their primarily specific microenvironments20.
MSCs’ ability to down-regulate major histocompatibility complex (MHC) class II makes them suitable for cell-based therapy without the risk of immune rejection21. In addition, their secretion of cytokines and growth factors with paracrine effects makes them ideal for regenerating damaged tissues22.
However, some challenges need to be considered for MSC therapy in clinical settings. Despite all their superior features, the heterogeneity of MSC populations has made it challenging to generalize the findings of different research groups since differences in culture conditions, donor, passage, and cell density affect the MSC phenotype23.
A safety issue relating to tumorigenicity concerns has recently posed a challenge to using MSCs for clinical applications. Tumors can develop due to spontaneous malignant transformation of MSCs after in vitro culturing or the immunosuppressive environment created by MSCs in vivo24. However, no evidence of tumor development has been reported in MSC-treated patients. Therefore, further investigation and monitoring of patients over longer follow-ups are essential to draw conclusions about the tumorigenicity potential of MSC therapy in clinical settings.
Another challenge of MSCs therapy, which is its primary limitation, is the potential of MSCs to be embolized and trigger clotting in the microvasculature, leading to impaired homing capacity. In some clinical studies, cases of pulmonary embolism have been observed in patients who had been given multiple intravenous MSC infusions25, 26. To meet this challenge, various protocols based on anticoagulant therapy such as low-dose heparin have been used in various studies26.
The role of different MSCs in restoring fertilization
While MSCs are traditionally isolated from bone marrow, they have also been found in many other adult tissues in recent years, such as bone marrow (BMSCs), adipose tissue (ADSCs), endometrial tissue, menstrual blood (MenSCs), umbilical cord (UCMSCs), amnion (AMSCs), and placenta (PMSCs)18.
BMSCs
BMSCs are primarily present in the bone marrow microenvironment and are a type of adult stem cell. These stem cells have multipotent differentiation potential with low immunogenicity27. Under certain conditions, BMSCs can differentiate into various tissue cells, such as adipocytes and bone cartilage, and also self-renew28. BMSCs are easily isolated and proliferated in vitro and can also migrate to damaged tissue29. Due to their immunomodulatory and paracrine features, BMSCs are believed to have therapeutic potential for infertility30. Studies have shown that cytokines-induced BMSCs can migrate to the damaged tissue and then secrete definite cytokines31. Some of these anti-fibrosis and anti-apoptosis cytokines, such as insulin-like growth factor (IGF), vascular endothelial growth factor (VEGF), and hepatocyte growth factor (HGF), help ovarian restoration30. Furthermore, anti-inflammatory and anti-oxidative cytokines such as interleukin (IL)-6 secreted by BMSCs can protect ovarian function31.
In vivo studies on ovaries indicated that BMSCs are helpful in treating animal models with premature ovarian failure (POF). In a mouse model with POF, BMSCs reactivated ovarian hormone production and folliculogenesis that had been damaged by chemotherapy32. Another study in rats suggests BMSCs can decrease perimenopause- and cisplatin-induced apoptosis in granulosa cells33. In humans, a clinical trial showed that autologous BMSCs might improve POF conditions in patients with idiopathic POF30.
Animal studies and clinical trials indicate successful treatment of endometrial dysfunction with BMSCs. Animal studies have shown that injection of BMSCs resulted in the secretion of various growth factors into the endometrium that can strongly stimulate cell proliferation and differentiation in the microvascular endothelium34, 35, 36. Moreover, in a mouse model with a thin endometrium, BMSC transplantation upregulated endometrial receptivity markers and improved infertility37. In a rat model, injection of BMSCs into the uterine cavity caused high expression of endometrial cell markers, resulting in increased endometrial thickness38. Several studies have shown that BMSCs migrate to the endometrium after systemic infusion. Studies in a mouse model showed that BMSCs migrate from donor male bone marrow into female recipients’ uterus via systemic infusion. For example, immunofluorescence and fluorescent in situ hybridization were performed on the uterus of female mice receiving BMSC transplantations from males. Then, the migration of BMSCs was confirmed by detecting the Y chromosome and the sex-determining region of Chr Y (Sry) gene in the uterus of female mice39. Moreover, a clinical trial on women with bone marrow transplants detected donor-derived cells in the uterine tissue, confirming the migration of BMSCs40.
In AS (IUAs and/or intracervical adhesions), BMSC transplantation effectively repaired endometrium damage by upregulating the expression of the estrogen (ER) and progesterone (PR) receptors41. In a rat model with endometrial cavity fibrosis, BMSC injection improved reproductive function by restoring endometrial receptivity and lining35. Clinical studies on AS suggest that the transplantation of prominin 1 (PROM1/CD133)+ BMSCs into patients can cause endometrial regeneration42. BMSC infusion improved reproductive function in patients by enhancing endometrial vascular density and refining the intensity and duration of menstruation. Surprisingly, some patients became pregnant after treatment without medical intervention42.
Besides these positive effects and features, there are some limitations to using BMSCs, including the need for an invasive procedure to isolate them, their low proliferation capacity (due to a low number of MSCs in the bone marrow), and their potential to differentiate into undesirable cell types with increasing donor age43, 44 (Table 1).
MSC type | Priorities | Limitations | Disorder/Clinical trials |
---|---|---|---|
Bone Marrow Mesenchymal Stem Cells | -Best known source -High angiogenic potential (stable during cell passages ) -High secretion of IL-6 -Strong antibacterial properties | -Requires invasive procedure to isolate -Low cell proliferation capacity - Differentiation potential with increasing donor age | Premature ovarian failure (NCT04815213), (NCT02062931) Asherman syndrome (NCT02144987) Atrophic Endometrium (NCT03166189) Azoospermia (NCT02025270) |
Adipose-Derived Mesenchymal Stem Cells |
-High angiogenic potential (Low passage cells
- Requires relatively invasive
procedures to collect compared to MSCs from neonatal tissues,
-Lower survival and quantity after liposuction
|
Premature ovarian failure (NCT02603744)
|
|
Menstrual Blood-Derived Mesenchymal Stem Cells | -Easy accessible -High proliferative capacity low immunogenicity -Anti tumor effect | Need to more research | Severe Asherman’s syndrome (ChiCTR-ONB-15007464) |
Umbilical Cord Mesenchymal Stem Cells | -Do not require invasive procedures to collect -Strong immunomodulatory properties -High proliferation rate than AT- and BM-MSC -Lack of differentiation toward adipocytes -Antitumor effects | -Limited collection at birth -Low efficiency of isolation - Heterogeneous cell population with different characteristics | Premature ovarian failure (NCT02644447) Primary Ovarian Insufficiency (NCT03033277) Asherman syndrome (NCT02313415) |
Amnion-Derived Mesenchymal Stem Cells | -Do not require invasive procedures to collect -Strong immunomodulatory properties -Angiogenesis properties | -Reduced numbers with aging -Restricted expansion in vitro | |
Placenta Mesenchymal Stem Cells (PMSCs) | -Do not require invasive procedures to collect -Higher homogeneous and primitive population -High proliferative rate -More immunosupressive -Less immunogenic -Less adipogenic potential -Antitumor effects -Younger cells that exposed less time to harmful agents -Higher homing capacity | Need to more research | Peyronie's Disease (NCT02395029) Erectile Dysfunction (NCT02398370) |
ADSCs
The general characteristics of MSCs, such as self-renewal, immunomodulation, and differentiation, are also seen in ADSCs, which are isolated from adipose tissue, and due to their ease of extraction through liposuction, have been intensively used in therapy38. Several animal studies have shown that ADSCs could be used to treat infertility45. One animal study showed the positive effect of ADSC injection on the viability of ovarian follicles. ADSCs increased the maintenance of grafts in the ovary and improved graft efficacy46, 47, 48. For example, intraperitoneal injection of ADSCs in mice improved ovarian function in chemotherapy-damaged ovaries47. Similarly, ADSCs improved ovarian dysfunction in rat models, increasing the rate of maturing follicles, oocyte number, and corpora lutea by altering the gene expression and secretion of specific paracrine cytokines46. Furthermore, ADSC treatment decreased apoptosis in granulosa cells. Therefore, ADSCs could be an alternative approach for POF therapy that could be useful in clinical applications and regenerative medicine47.
ADSCs can also improve fertility in animals by increasing endometrial thickness and the number of endometrial glands and microvessels. Furthermore, since ADSCs can differentiate into endometrial cells, their transplantation can repair the endometrial injury. Treating rat models with ADSCs and estrogen restored endometrial tissue, proved via detecting green fluorescent protein (GFP) in endometrial epithelial cells grafted with GFP-labeled ADSCs49, 50. A clinical trial using autologous ADSCs to repair the endometrium recorded the first successful pregnancies and childbirths. Its results showed that endometrium thickness increased in 20 out of 25 patients after sub-endometrial injection of ADSCs. Thirteen women became pregnant, and nine successful childbirths were recorded51.
Regarding male infertility, ADSCs helped restore fertility and sperm production in rats with azoospermia52. In humans, an in vitro study showed that the supernatant product of ADSCs could restore sperm motility in infertile male patients. This effect appeared due to growth factors and bioactive molecules positively affecting sperm motility53. Furthermore, intracytoplasmic injection of sperm showed that paracrine factors in the medium isolated from ADSCs could result in oocyte maturation and embryo formation54.
As mentioned above, ADSCs are a promising source for therapeutic applications because of their ease of isolation and availability in high frequency through liposuction. However, ADSCs are embedded in a complex niche and interact with other factors and cells. After their isolation via liposuction and separation from their niche, ADSC features such as proliferation capacity are reduced, possibly due to local anesthesia, which can negatively impact their survival and quantity43, 55, 56, 57 (Table 1).
MenSCs
Since the need for surgery complicates MSC isolation from bone marrow, adipose tissue, or amniotic fluid, it was necessary to find more accessible alternative sources of MSCs58. Recent studies have shown that endometrial basal layer cells have stem cell characteristics such as self-renewal and proliferation59. Endometrial stem cells are new objects in treating infertility (of unclear origin) in women. However, a biopsy or cuvette to access the endometrial stem cells may harm the endometrium60. Interestingly, MenSCs are functionally and morphologically similar to endometrial stem cells. MenSCs match the International Society for Cell and Gene Therapy criteria for MSC characteristics and express dual ESC and MSC markers61. They proliferate twice as fast and regenerate better than BMSCs and MSCs. This proliferation rate is superior for clinical use because when many stem cells can be quickly isolated from the same source, it is a noninvasive, painless procedure with no ethical issues62, 63.
MenSCs can be used as ideal MSCs for treating endometrial infertility. In a nude mouse model with endometrial damage, MenSCs survived in the endometrium after transplantation38. Furthermore, they improved the rate of embryo implantation by upregulating the levels of keratin, vimentin, and VEGF in the endometrium and increasing endometrial thickness by regulating a protein kinase B (PKB/AKT)-related signaling pathway38, 64 because this kinase plays an important role in cell survival, proliferation, and metabolism65.
A clinical trial on patients with severe AS reported promising results after the transplantation of autologous MenSCs. They showed that MenSCs increased endometrial thickness and improved the rate and quality of pregnancy in patients with AS66. Unlike BMSCs, AMSCs, and UCMSCs, MenSCs have shown no limitations in accessibility, collection, proliferation rate in vitro, or heterogeneity. However, further basic and clinical studies are needed (Table 1).
UCMSCs
Human UCMSCs (hUCMSCs), also called Wharton jelly MSCs, can be obtained from newborns’ cord tissue. HUCMSCs have stem cell characteristics such as high proliferation, differentiation potency, low immunogenicity, and prolonged survival time after transplantation67.
Since hUCMSCs are safe and effective, their use has been considered to treat infertility. HUCMSCs repaired damaged endometrium in an animal model68. They also increased the rate of implanted embryos by upregulating vascular and downregulating pro-inflammatory factors69. It has also been shown that uterine niches formed in animals after a cesarean could be effectively treated by intramuscular injection of hUCMSCs70. In clinical trials on patients with IUA, hUCMSC-loaded biodegradable collagen scaffolds have been safely and efficiently implanted into the patient’s uterine cavity. After transplantation, the survival of the hUCMSCs was maintained at the site of endometrial damage57. These results indicate a hopeful future for IUA therapy and demonstrate that tissue engineering with hUCMSCs can increase their therapeutic efficiency71.
Besides the positive characteristics of UCMSCs, they have disadvantages such as limited collection at birth (attributed to uncertainty about the baby’s health), low isolation efficiency, and high heterogeneity72, 73, 74 (Table 1).
AMSCs
The amnion is usually discarded as medical waste after delivery. The amniotic tissue, a postpartum membrane, is a rich resource of human MSCs called AMSCs (hAMSCs)75. HAMSCs have some advantages over MSCs. Besides MSC properties, they also have some ESC phenotypic properties. In addition, the methods used to isolate hAMSCs are noninvasive, safe, and without ethical issues. These superior properties make hAMSCs a good target for regenerative medicine76, 69.
In the chemotherapy-induced POI rat model, hAMSC transplantation lessened ovarian injury and improved ovarian function77. In an IUA rat model, hAMSC transplantation downregulated pro-inflammatory cytokines such as IL-1β and tumor necrosis factor-α (TNF-α) and upregulated anti-inflammatory cytokines such as IL-6 and basic fibroblast growth factor (bFGF)78. These results show that hAMSC transplantation could improve endometrial restoration, likely due to their immunomodulatory characteristics79.
In the mouse model of age-related diminished ovarian reserve, hAMSC transplantation improved ovarian function and oocyte maturation by modifying the ovarian microenvironment through the protein kinase, AMP-activated, alpha 2 catalytic subunit (PRKAA2/AMPK)/forkhead box O3 (FOXO3/FOXO3A) signaling pathway80.
Interestingly, the epithelial cells derived from amniotic tissue, called human amniotic epithelial cells (hAECs), also exhibit stem cell features and have the potential for cell-based therapy. Like hAMSCs and all MSCs, hAECs have the potential for multipotential differentiation, immune regulation, and low tumorigenicity81. HAEC transplantation improved endometrial morphology in IUA mouse models, possibly by stimulating endometrial stromal cells’ proliferation and angiogenesis and increasing endometrium thickness58. Moreover, the transplanted hAECs triggered endometrium autophagy in the IUA mouse models, reducing the fibrotic region of the endometrium and thereby improving infertility.
Finally, the most important limitations of AMSCs include restricted pluripotency, reduced numbers with aging, and limited expansion in vitro72, 82 (Table 1).
PMSCs
The placenta is a temporary organ made with the participation of fetal and maternal tissues that is excreted after delivery. A population of MSCs can be isolated from the human placenta, called PMSCs83. This tissue is easily accessible (noninvasive) and abundant. It has immunoregulating, self-renewing, and differentiating properties. PMSCs are not donor-age-dependent and express common BMSC markers. Due to their properties, PMSCs are an attractive source of MSCs for stem cell-based therapy84.
PMSCs have been shown to secrete several cytokines, including colony-stimulating factor 3 (CSF3/G‐CSF), IL‐6/‐8/‐10, and C-C motif chemokine ligand 5 (CCL5/RANTES)85. The secretion of KIT ligand (KITLG/SCF) after PMSC transplantation promoted oocyte survival, elevating the expression levels of lin-28 homolog A (Lin28a), LIM homeobox protein 8 (Lhx8), NOBOX oogenesis homeobox (Nobox), and nanos C2HC-type zinc finger 3 (Nanos3), thereby improving ovarian function67. These findings suggest that PMSC therapy could be used to treat individuals with infertility and ovarian dysfunction, such as PCOS67.
Two clinical trials by Levy et al. evaluated the efficacy and safety of PMSCs in treating men with Peyronie’s disease86 and erectile dysfunction (ED)87, reporting beneficial results as a nonsurgical treatment. These two diseases do not inherently cause infertility. However, reports suggest that since these diseases may affect the strength and completeness of ejaculation, they can affect a man’s ability to conceive88.
However, most clinical trials on PMSCs are just at the beginning of the path, do not yet have published results, and require further research89.
Stem cells’ paracrine mechanism
Extracellular vesicles derived from MSCs
Stem cells are widely used in reproductive medicine and have direct and indirect (paracrine, such as cytokines) therapeutic effects90. However, due to the limitations of injecting living cells, it is likely better to use their paracrine elements, such as extracellular vesicles and microRNAs (miRNAs). Exosomes are a well-characterized subset of extracellular vesicles that are secreted by MSCs. They encompass various cellular compounds, including lipids, proteins, coding and non-coding RNAs (mRNAs, tRNAs, and miRNAs), DNA fragments, and cell surface proteins91. The compounds present in various exosomes differ based on the origin of the MSCs92. Since exosomes are important carrier organelles for intercellular cross-talk, they play an important role in many physiological functions93.
Specific surface molecules on exosomes facilitate their interactions with their target cells. They include MHC I and II molecules, galectin, integrin, intercellular adhesion molecule 1 (ICAM1), and collagen94. Exosomes can trigger cell migration, proliferation, viability, angiogenesis, oogenesis, spermatogenesis, and acrosome reaction. They can alter different signaling pathways and are involved in gene expression, immune system downregulation, and embryo implantation induction95. Exosomes have an important effect on the reproductive mechanism and can be used to treat reproductive diseases such as POI and ED95. In POI models, transplantation of hAMSC-derived exosomes decreased apoptosis in tissue grafts by upregulating SMAD family member 5 (SMAD5) expression to downregulate caspase-3 (CASP3), caspase-8 (CASP8), and Fas cell surface death receptor (FAS)/Fas ligand (FASLG/FasL)96. HAMSC-derived exosomes also upregulate SMAD family members 2 (SMAD2) and 3 (SMAD3), enhancing granulosa cell (GC) proliferation, activating ovulation, and producing corpus luteum.
It has been suggested that an exosome combination from several sources (MSCs, hUCMSCs, and bovine granulosa) may be effective for treating asthenozoospermia because it could reduce the reactive oxygen rate97. In addition, combining exosomes from retinal astroglial, cardiomyocytes, and MSCs was suitable for treating endometriosis in male rats with type 2 diabetes. Combining these exosomes likely inhibited induced cell death, macrophage infiltration, and angiogenesis in the ectopic endometrium98, 99, 100.
Regarding mouse mating, tetraspanin proteins (e.g., CD81 and CD9) vital for oocyte fertilization are produced by oocyte-derived exosomes, and oocytes produced by mice lacking these proteins cannot fuse with sperm101, 102. Placenta-derived exosomes are important in decreasing T regulatory cells and downregulating the maternal immune system during gestation103, 104, 105 by secretion of MHC-I, MHC-II, UL16 binding protein 1–5 (ULBP1–5), and FASLG105.
Overall, using exosomes is less risky and more feasible than stem cells because they protect their contents from damaging enzymes, do not induce inflammation, and do not generate teratomas105.
The role of microRNAs in driving MSC therapeutic outcomes
Most of the therapeutic effects of MSCs are due to paracrine producers, especially through the release of soluble factors such as miRNAs and exosomal miRNAs. In other words, miRNAs mediate the effects of MSCs and are therefore considered new therapeutic factors106.
Studies have shown that miRNAs inhibit target mRNA translation and thereby have a critical effect on regulating stem cell differentiation and regeneration107. The miRNAs may show similar effects in regulating stem cells’ pathological and physiological features related to ovarian function. In female rats with cyclophosphamide-induced POF, GC apoptosis was associated with the upregulation of miR-21 in BMSCs108. GCs are somatic steroidogenic cells surrounding the oocytes and are essential for developing oocytes since they generate nutrients and growth factors109. It has been shown that a high miR-21 level in BMSCs reduces their expression of programmed cell death protein 4 (PDCD4), inducing GC apoptosis and leading to abnormal oocyte development110. In addition, miR-21 overexpression in BMSCs may increase estradiol and decrease follicle-stimulating hormone levels108.
Besides cytoplasmic miRNAs, exosomal miRNAs can modulate intercellular signal transduction and regulate molecular mechanisms in various diseases111. Two studies have shown the role of some BMSC-derived exosomal miRNAs in activating the restoration of ovarian function in an animal model of POF4. BMSC-derived exosomal miR-644-5p regulated tumor protein p53 (TP53) signaling, inhibiting GC death112. BMSC-derived exosomal miR-144-5p regulated phosphatase and tensin homolog (PTEN) in rats with chemotherapy-induced POF, restoring ovarian function. These findings show that gene expression regulation by miRNAs could be a subset of BMSC-based therapy113.
Co-transplantation of MSCs with other elements
The effectiveness of transplantation protocols combined with other factors was examined in a rat model. Treating IUA with MenSCs combined with platelet-rich plasma (PRP) improved proliferation and angiogenesis and morphologically restored the endometrium108. In addition, combining estrogen with ADSCs induced endometrial tissue regeneration in a rat model38. Co-transplantation of PRP and MSCs into an animal model of POI increased IGF-1 and transforming growth factor (TGF)-β levels and C-X-C motif chemokine ligand 12 (CXCL12) expression (an anti-inflammatory chemokine). Indeed, PRP reduced inflammatory responses and the extent and number of follicular atresia114. Applying a collagen scaffold with ADSCs in preclinical experiments on rats with POF led to long-term ADSC maintenance in their ovaries. This long duration improved ovarian function and fertility restoration in rats115. Co-transplanting spermatogonial stem cells with TGF-ß1-treated MSCs in mice improved fertility efficiency because TGF-ß1 treatment preferentially conducts MSCs to the testis where their secretion elements could recover the testicular niche116.
Delivery of MSCs to treat infertility-related disorders in clinical trials
There have been tried and tested approaches in clinical trials to deliver MSCs to the reproductive system: (1) intra-tissue injection, (2) tissue intra-arterial injection, and (3) an MSC-loaded collagen scaffold.
Most clinical trials have used intra-tissue injections. MSCs have been transplanted into reproductive system organs of women with POF under the guidance of transvaginal sonography/ultrasound (NCT03033277, NCT02603744, NCT04815213, and NCT04815213) or via laparoscopy (NCT02062931). In women with atrophic endometrium and AS, it has been conducted through the uterine cervix under ultrasound guidance (NCT03166189 and ChiCTR-ONB-15007464). MSCs have been injected into the rete testis of men with azoospermia using a special syringe (NCT02025270). MSCs have also been intracavernosally and intralesionally injected into men with Peyronie’s disease and ED (NCT02395029 and NCT02398370).
One clinical trial using MSCs to treat AS and endometrial atrophy transplanted them into the spiral arterioles of the uterus via tissue intra-arterial injection to regenerate the endometrium de novo (NCT02144987).
Other clinical trials have examined the safety and efficiency of an approach combining a collagen scaffold and MSCs. MSC-loaded collagen scaffolds have been transplanted into the uterine cavity during hysteroscopy of women with a thin or scarred endometrium (NCT03592849) and with IUAs (NCT02313415). Another clinical trial used this approach, giving women with POF a bilateral ovarian injection of MSCs with an injectable collagen scaffold (NCT02644447).
As described above, all clinical trials on MSC therapies for infertility have used local delivery methods. Those clinical trials did not explore the systemic delivery of MSCs through intravenous injection. One of the strongest justifications for this is that given the main challenge of MSCs —their potential to trigger clotting in microvasculature leading to impaired homing capacity—intravenous injection of MSCs could make the treatment process inefficient and challenging.
Conclusions
In summary, infertility is a medical/social problem affecting many couples worldwide. Therefore, this review offers a greater understanding of the role of MSCs in infertility treatment and their limitations, which should be considered before using MSCs in clinical trials. Therefore, the appropriate MSC source must be selected according to the priorities and limitations of each MSC type and the therapeutic purpose (tissue regeneration, paracrine pathway-like immunomodulation, or both) in the reproductive system.
It appears that systemic intravascular delivery of MSCs from various sources could be more challenging and inefficient than local delivery due to the limitation of MSCs in clot formation, leading to impaired homing capacity.
Abbreviations
AD-MSCs: Amnion-Derived Mesenchymal Stem Cells, AR: Androgen receptor mutations, ART: Assisted reproductive technology, BMSCs: Bone Marrow Stem Cells; ER and PR: Estrogen and progesterone receptor; FSH: Follicle-stimulating hormone; GCs: Granulosa cells; HADMSC-Exos: Exosomes derived from human adipose mesenchymal stem cells; HGF: Hepatocyte growth factor; hAECs: Human amniotic epithelial cells; hAMSCs: Human amniotic-derived stem cells; hUC-MSCs: Human umbilical cord-derived stem cells; IGF: Insulin-like growth factor; ICAM: Intercellular adhesion molecules; IL-6: Interleukin-6; IUA: Intrauterine adhesions; MHC: Major histocompatibility complex; MenSCs: Menstrual Blood-Derived Mesenchymal Stem Cells; MSCs: Mesenchymal stem cells; PCOS: Polycystic ovaries; PTEN: Phosphatase and tensin homolog; PMSCs: Placental Mesenchymal Stem Cells; PRP: Platelet-rich plasma; POI: Premature ovarian insufficiency; PDCD4: Programmed cell death protein 4; SMAD2: Small mothers against decapentaplegic; SSCs: Spermatogonial stem cells; SPAS: Supernatant product of ADSCs; T reg: T regulatory; ULBP1: UL16-binding protein 1; VEGF: Vascular endothelial growth factor
Acknowledgments
The authors thank the staff of the Hematopoietic Stem Cell Research Center of Shahid Beheshti University of Medical Sciences for providing the possibility of doing the study and for useful assistance.
Author’s contributions
R.M., M.A., E.R., M.S. conception and design, acquisition of data; R.M., M.V.L., M.M., M.R.M.,D.H.,:drafting the article or revising it critically for important intellectual content. All authors revised final approval of the version to be submitted for publication.
Funding
None.
Availability of data and materials
Not applicable.
Ethics approval and consent to participate
Not applicable.
Consent for publication
Not applicable.
Competing interests
The authors declare that they have no competing interests.
References
-
Chang
Z.,
Zhu
H.,
Zhou
X.,
Zhang
Y.,
Jiang
B.,
Li
S.,
Mesenchymal Stem Cells in Preclinical Infertility Cytotherapy: A Retrospective Review. Stem Cells International.
2021;
2021
:
8882368
.
View Article Google Scholar -
Thoma
M.,
Fledderjohann
J.,
Cox
C.,
Adageba
R.K.,
Biological and social aspects of human infertility: a global perspective. Oxford Res Encycl Glob Public Health..
2021;
:
1-73
.
View Article Google Scholar -
Ru
F.,
Application of Stem Cell Therapy. Zhongguo Linchuang Kangfu.
2006;
10
(5)
:
133-5
.
-
Esfandyari
S.,
Chugh
R.M.,
Park
H.S.,
Hobeika
E.,
Ulin
M.,
Al-Hendy
A.,
Mesenchymal stem cells as a bio organ for treatment of female infertility. Cells.
2020;
9
(10)
:
2253
.
View Article Google Scholar -
Liao
Z.,
Liu
C.,
Wang
L.,
Sui
C.,
Zhang
H.,
Therapeutic role of mesenchymal stem cell-derived extracellular vesicles in female reproductive diseases. Frontiers in Endocrinology (Lausanne).
2021;
12
:
665645
.
View Article Google Scholar -
Skakkebaek
N.E.,
Meyts
E. Rajpert-De,
Main
K.M.,
Testicular dysgenesis syndrome: an increasingly common developmental disorder with environmental aspects. Human Reproduction (Oxford, England).
2001;
16
(5)
:
972-8
.
View Article Google Scholar -
Bhartiya
D.,
Hinduja
I.,
Patel
H.,
Bhilawadikar
R.,
Making gametes from pluripotent stem cells - a promising role for very small embryonic-like stem cells. Reproductive Biology and Endocrinology.
2014;
12
(1)
:
114
.
View Article Google Scholar -
Ferlin
A.,
Raicu
F.,
Gatta
V.,
Zuccarello
D.,
Palka
G.,
Foresta
C.,
Male infertility: role of genetic background. Reproductive Biomedicine Online.
2007;
14
(6)
:
734-45
.
View Article Google Scholar -
O'Brien
K.L. O'Flynn,
Varghese
A.C.,
Agarwal
A.,
The genetic causes of male factor infertility: a review. Fertility and Sterility.
2010;
93
(1)
:
1-12
.
View Article Google Scholar -
Salazar
C.A.,
Isaacson
K.,
Morris
S.,
A comprehensive review of Asherman's syndrome: causes, symptoms and treatment options. Current Opinion in Obstetrics & Gynecology.
2017;
29
(4)
:
249-56
.
View Article Google Scholar -
Lo
B.,
Parham
L.,
Ethical issues in stem cell research. Endocrine Reviews.
2009;
30
(3)
:
204-13
.
View Article Google Scholar -
Khan
F.A.,
Almohazey
D.,
Alomari
M.,
Almofty
S.A.,
Isolation, culture, and functional characterization of human embryonic stem cells: current trends and challenges. Stem Cells International.
2018;
2018
:
1429351
.
View Article Google Scholar -
Fazeli
Z.,
Abedindo
A.,
Omrani
M.D.,
Ghaderian
S.M.,
Mesenchymal stem cells (MSCs) therapy for recovery of fertility: a systematic review. Stem Cell Reviews and Reports.
2018;
14
(1)
:
1-12
.
View Article Google Scholar -
Han
Y.,
Li
X.,
Zhang
Y.,
Han
Y.,
Chang
F.,
Ding
J.,
Mesenchymal stem cells for regenerative medicine. Cells.
2019;
8
(8)
:
886
.
View Article Google Scholar -
Zakrzewski
W.,
Dobrzyński
M.,
Szymonowicz
M.,
Rybak
Z.,
Stem cells: past, present, and future. Stem Cell Research & Therapy.
2019;
10
(1)
:
68
.
View Article Google Scholar -
Zhankina
R.,
Baghban
N.,
Askarov
M.,
Saipiyeva
D.,
Ibragimov
A.,
Kadirova
B.,
Mesenchymal stromal/stem cells and their exosomes for restoration of spermatogenesis in non-obstructive azoospermia: a systemic review. Stem Cell Research & Therapy.
2021;
12
(1)
:
229
.
View Article Google Scholar -
Christodoulou
I.,
Goulielmaki
M.,
Devetzi
M.,
Panagiotidis
M.,
Koliakos
G.,
Zoumpourlis
V.,
Mesenchymal stem cells in preclinical cancer cytotherapy: a systematic review. Stem Cell Research & Therapy.
2018;
9
(1)
:
336
.
View Article Google Scholar -
Rojewski
M.T.,
Weber
B.M.,
Schrezenmeier
H.,
Phenotypic characterization of mesenchymal stem cells from various tissues. Transfusion Medicine and Hemotherapy ; Offizielles Organ der Deutschen Gesellschaft fur Transfusionsmedizin und Immunhamatologie.
2008;
35
(3)
:
168-84
.
View Article Google Scholar -
Mizuno
M.,
Katano
H.,
Mabuchi
Y.,
Ogata
Y.,
Ichinose
S.,
Fujii
S.,
Specific markers and properties of synovial mesenchymal stem cells in the surface, stromal, and perivascular regions. Stem Cell Research & Therapy.
2018;
9
(1)
:
123
.
View Article Google Scholar -
Shammaa
R.,
El-Kadiry
A.E.,
Abusarah
J.,
Rafei
M.,
Mesenchymal stem cells beyond regenerative medicine. Frontiers in Cell and Developmental Biology.
2020;
8
:
72
.
View Article Google Scholar -
Musia\l-Wysocka
A.,
Kot
M.,
Majka
M.,
The pros and cons of mesenchymal stem cell-based therapies. Cell Transplantation.
2019;
28
(7)
:
801-12
.
View Article Google Scholar -
Baraniak
P.R.,
McDevitt
T.C.,
Stem cell paracrine actions and tissue regeneration. Regenerative Medicine.
2010;
5
(1)
:
121-43
.
View Article Google Scholar -
Ankrum
J.,
Karp
J.M.,
Mesenchymal stem cell therapy: Two steps forward, one step back.2010.. Trends in molecular medicine.
2010;
16
(5)
:
203-9
.
View Article Google Scholar -
Barkholt
L.,
Flory
E.,
Jekerle
V.,
Lucas-Samuel
S.,
Ahnert
P.,
Bisset
L.,
Büscher
D.,
Fibbe
W.,
Foussat
A.,
Kwa
M.,
Lantz
O.,
Risk of tumorigenicity in mesenchymal stromal cell–based therapies—bridging scientific observations and regulatory viewpoints. Cytotherapy.
2013;
15
(7)
:
753-9
.
View Article Google Scholar -
Jung
J.W.,
Kwon
M.,
Choi
J.C.,
Shin
J.W.,
Park
I.W.,
Choi
B.W.,
Familial occurrence of pulmonary embolism after intravenous, adipose tissue-derived stem cell therapy. Yonsei Medical Journal.
2013;
54
(5)
:
1293-6
.
View Article Google Scholar -
Moll
G.,
Ignatowicz
L.,
Catar
R.,
Luecht
C.,
Sadeghi
B.,
Hamad
O.,
Jungebluth
P.,
Dragun
D.,
Schmidtchen
A.,
Ringdén
O.,
Different procoagulant activity of therapeutic mesenchymal stromal cells derived from bone marrow and placental decidua. Stem cells and development.
2015;
24
(19)
:
2269-79
.
View Article Google Scholar -
Miao
C.,
Lei
M.,
Hu
W.,
Han
S.,
Wang
Q.,
A brief review: the therapeutic potential of bone marrow mesenchymal stem cells in myocardial infarction. Stem Cell Research & Therapy.
2017;
8
(1)
:
242
.
View Article Google Scholar -
Pittenger
M.F.,
Discher
D.E.,
Péault
B.M.,
Phinney
D.G.,
Hare
J.M.,
Caplan
A.I.,
Mesenchymal stem cell perspective: cell biology to clinical progress. NPJ Regenerative Medicine.
2019;
4
(1)
:
22
.
View Article Google Scholar -
Li
M.,
Ikehara
S.,
Bone-marrow-derived mesenchymal stem cells for organ repair. Stem Cells International.
2013;
2013
:
132642
.
View Article Google Scholar -
He
Y.,
Chen
D.,
Yang
L.,
Hou
Q.,
Ma
H.,
Xu
X.,
The therapeutic potential of bone marrow mesenchymal stem cells in premature ovarian failure. Stem Cell Research & Therapy.
2018;
9
(1)
:
263
.
View Article Google Scholar -
Fathi
E.,
Farahzadi
R.,
Valipour
B.,
Sanaat
Z.,
Cytokines secreted from bone marrow derived mesenchymal stem cells promote apoptosis and change cell cycle distribution of K562 cell line as clinical agent in cell transplantation. PLoS One.
2019;
14
(4)
:
e0215678
.
View Article Google Scholar -
Mohamed
S.A.,
Shalaby
S.M.,
Abdelaziz
M.,
Brakta
S.,
Hill
W.D.,
Ismail
N.,
Human mesenchymal stem cells partially reverse infertility in chemotherapy-induced ovarian failure. Reproductive Sciences (Thousand Oaks, Calif.).
2018;
25
(1)
:
51-63
.
View Article Google Scholar -
Guo
J.Q.,
Gao
X.,
Lin
Z.J.,
Wu
W.Z.,
Huang
L.H.,
Dong
H.Y.,
BMSCs reduce rat granulosa cell apoptosis induced by cisplatin and perimenopause. BMC Cell Biology.
2013;
14
(1)
:
18
.
View Article Google Scholar -
Abumaree
M.H.,
Jumah
M.A. Al,
Kalionis
B.,
Jawdat
D.,
Khaldi
A. Al,
AlTalabani
A.A.,
Phenotypic and functional characterization of mesenchymal stem cells from chorionic villi of human term placenta. Stem Cell Reviews and Reports.
2013;
9
(1)
:
16-31
.
View Article Google Scholar -
Gao
L.,
Huang
Z.,
Lin
H.,
Tian
Y.,
Li
P.,
Lin
S.,
Bone marrow mesenchymal stem cells (BMSCs) restore functional endometrium in the rat model for severe Asherman syndrome. Reproductive Sciences (Thousand Oaks, Calif.).
2019;
26
(3)
:
436-44
.
View Article Google Scholar -
Bhartiya
D.,
Singh
P.,
Sharma
D.,
Kaushik
A.,
Very small embryonic-like stem cells (VSELs) regenerate whereas mesenchymal stromal cells (MSCs) rejuvenate diseased reproductive tissues. Stem Cell Reviews and Reports.
2021;
\textbullet\textbullet\textbullet
:
1-0
.
View Article Google Scholar -
Yi
K.W.,
Mamillapalli
R.,
Sahin
C.,
Song
J.,
Tal
R.,
Taylor
H.S.,
Bone marrow-derived cells or C-X-C motif chemokine 12 (CXCL12) treatment improve thin endometrium in a mouse model. Biology of Reproduction.
2019;
100
(1)
:
61-70
.
View Article Google Scholar -
Rungsiwiwut
R.,
Virutamasen
P.,
Pruksananonda
K.,
Mesenchymal stem cells for restoring endometrial function: an infertility perspective. Reproductive Medicine and Biology.
2020;
20
(1)
:
13-9
.
View Article Google Scholar -
Du
H.,
Taylor
H.S.,
Contribution of bone marrow-derived stem cells to endometrium and endometriosis. Stem Cells (Dayton, Ohio).
2007;
25
(8)
:
2082-6
.
View Article Google Scholar -
Taylor
H.S.,
Endometrial cells derived from donor stem cells in bone marrow transplant recipients. Journal of the American Medical Association.
2004;
292
(1)
:
81-5
.
View Article Google Scholar -
Wang
J.,
Ju
B.,
Pan
C.,
Gu
Y.,
Zhang
Y.,
Sun
L.,
Application of bone marrow-derived mesenchymal stem cells in the treatment of intrauterine adhesions in rats. Cellular Physiology and Biochemistry.
2016;
39
(4)
:
1553-60
.
View Article Google Scholar -
Santamaria
X.,
Cabanillas
S.,
Cervelló
I.,
Arbona
C.,
Raga
F.,
Ferro
J.,
Autologous cell therapy with CD133+ bone marrow-derived stem cells for refractory Asherman's syndrome and endometrial atrophy: a pilot cohort study. Human Reproduction (Oxford, England).
2016;
31
(5)
:
1087-96
.
View Article Google Scholar -
Kozlowska
U.,
Krawczenko
A.,
Futoma
K.,
Jurek
T.,
Rorat
M.,
Patrzalek
D.,
Similarities and differences between mesenchymal stem/progenitor cells derived from various human tissues. World Journal of Stem Cells.
2019;
11
(6)
:
347-74
.
View Article Google Scholar -
Kern
S.,
Eichler
H.,
Stoeve
J.,
Klüter
H.,
Bieback
K.,
Comparative analysis of mesenchymal stem cells from bone marrow, umbilical cord blood, or adipose tissue. Stem Cells (Dayton, Ohio).
2006;
24
(5)
:
1294-301
.
View Article Google Scholar -
Fouad
H.,
Sabry
D.,
Elsetohy
K.,
Fathy
N.,
Therapeutic efficacy of amniotic membrane stem cells and adipose tissue stem cells in rats with chemically induced ovarian failure. Journal of Advanced Research.
2016;
7
(2)
:
233-41
.
View Article Google Scholar -
Omar
F.,
Amin
N.,
Elsherif
H.,
Mohamed
D.,
Role of adipose-derived stem cells in restoring ovarian structure of adult albino rats with chemotherapy-induced ovarian failure: a histological and immunohistochemical study. Journal of Carcinogenesis & Mutagenesis.
2016;
7
(254)
:
2
.
View Article Google Scholar -
Sun
M.,
Wang
S.,
Li
Y.,
Yu
L.,
Gu
F.,
Wang
C.,
Adipose-derived stem cells improved mouse ovary function after chemotherapy-induced ovary failure. Stem Cell Research & Therapy.
2013;
4
(4)
:
80
.
View Article Google Scholar -
Terraciano
P.,
Garcez
T.,
Ayres
L.,
Durli
I.,
Baggio
M.,
Kuhl
C.P.,
Cell therapy for chemically induced ovarian failure in mice. Stem Cells International.
2014;
2014
:
720753
.
View Article Google Scholar -
Zhao
S.,
Qi
W.,
Zheng
J.,
Tian
Y.,
Qi
X.,
Kong
D.,
Exosomes derived from adipose mesenchymal stem cells restore functional endometrium in a rat model of intrauterine adhesions. Reproductive Sciences (Thousand Oaks, Calif.).
2020;
27
(6)
:
1266-75
.
View Article Google Scholar -
Shao
X.,
Ai
G.,
Wang
L.,
Qin
J.,
Li
Y.,
Jiang
H.,
Adipose-derived stem cells transplantation improves endometrial injury repair. Zygote (Cambridge, England).
2019;
27
(6)
:
367-74
.
View Article Google Scholar -
Chen
G.,
Jin
Y.,
Shi
X.,
Qiu
Y.,
Zhang
Y.,
Cheng
M.,
Adipose-derived stem cell-based treatment for acute liver failure. Stem Cell Research & Therapy.
2015;
6
(1)
:
40
.
View Article Google Scholar -
Cakici
C.,
Buyrukcu
B.,
Duruksu
G.,
Haliloglu
A.H.,
Aksoy
A.,
Is\ik
A.,
Recovery of fertility in azoospermia rats after injection of adipose-tissue-derived mesenchymal stem cells: the sperm generation. BioMed Research International.
2013;
2013
:
529589
.
View Article Google Scholar -
Qomi
R. Tabatabaei,
Sheykhhasan
M.,
Adipose-derived stromal cell in regenerative medicine: A review. World Journal of Stem Cells.
2017;
9
(8)
:
107-17
.
View Article Google Scholar -
Ghiasi
M.,
Fazaely
H.,
Asaii
E.,
Sheykhhasan
M.,
In Vitro Maturation of Human Oocytes using Conditioned Medium of Mesenchymal Stem Cells and Formation of Embryo by Use of ICSI. Vitro..
2014;
41
(11)
:
978-85
.
-
Yarak
S.,
Okamoto
O.K.,
Human adipose-derived stem cells: current challenges and clinical perspectives. Anais Brasileiros de Dermatologia.
2010;
85
(5)
:
647-56
.
View Article Google Scholar -
Costa
L.A.,
Eiro
N.,
Fraile
M.,
Gonzalez
L.O.,
Saá
J.,
Garcia-Portabella
P.,
Functional heterogeneity of mesenchymal stem cells from natural niches to culture conditions: implications for further clinical uses. Cellular and Molecular Life Sciences.
2021;
78
(2)
:
447-67
.
View Article Google Scholar -
Cao
Y.,
Sun
H.,
Zhu
H.,
Zhu
X.,
Tang
X.,
Yan
G.,
Allogeneic cell therapy using umbilical cord MSCs on collagen scaffolds for patients with recurrent uterine adhesion: a phase I clinical trial. Stem Cell Research & Therapy.
2018;
9
(1)
:
192
.
View Article Google Scholar -
Li
B.,
Zhang
Q.,
Sun
J.,
Lai
D.,
Human amniotic epithelial cells improve fertility in an intrauterine adhesion mouse model. Stem Cell Research & Therapy.
2019;
10
(1)
:
257
.
View Article Google Scholar -
Tempest
N.,
Maclean
A.,
Hapangama
D.K.,
Endometrial stem cell markers: current concepts and unresolved questions. International Journal of Molecular Sciences.
2018;
19
(10)
:
3240
.
View Article Google Scholar -
Morelli
S.S.,
Yi
P.,
Goldsmith
L.T.,
Endometrial stem cells and reproduction. Obstetrics and Gynecology International.
2012;
2012
:
851367
.
View Article Google Scholar -
Bozorgmehr
M.,
Gurung
S.,
Darzi
S.,
Nikoo
S.,
Kazemnejad
S.,
Zarnani
A.H.,
Endometrial and menstrual blood mesenchymal stem/stromal cells: biological properties and clinical application. Frontiers in Cell and Developmental Biology.
2020;
8
:
497
.
View Article Google Scholar -
Chen
L.,
Qu
J.,
Xiang
C.,
The multi-functional roles of menstrual blood-derived stem cells in regenerative medicine. Stem Cell Research & Therapy.
2019;
10
(1)
:
1-10
.
View Article PubMed Google Scholar -
Lv
H.,
Hu
Y.,
Cui
Z.,
Jia
H.,
Human menstrual blood: a renewable and sustainable source of stem cells for regenerative medicine. Stem Cell Research & Therapy.
2018;
9
(1)
:
325
.
View Article Google Scholar -
Hu
J.,
Song
K.,
Zhang
J.,
Zhang
Y.,
Tan
B.Z.,
Effects of menstrual blood-derived stem cells on endometrial injury repair. Molecular Medicine Reports.
2019;
19
(2)
:
813-20
.
View Article Google Scholar -
Hemmings
B.A.,
Restuccia
D.F.,
PI3K-PKB/Akt pathway. Cold Spring Harbor Perspectives in Biology.
2012;
4
(9)
:
a011189
.
View Article Google Scholar -
Tan
J.,
Li
P.,
Wang
Q.,
Li
Y.,
Li
X.,
Zhao
D.,
Autologous menstrual blood-derived stromal cells transplantation for severe Asherman's syndrome. Human Reproduction (Oxford, England).
2016;
31
(12)
:
2723-9
.
View Article Google Scholar -
Choi
J.H.,
Seok
J.,
Lim
S.M.,
Kim
T.H.,
Kim
G.J.,
Microenvironmental changes induced by placenta-derived mesenchymal stem cells restore ovarian function in ovariectomized rats via activation of the PI3K-FOXO3 pathway. Stem Cell Research & Therapy.
2020;
11
(1)
:
486
.
View Article Google Scholar -
Xie
Q.,
Liu
R.,
Jiang
J.,
Peng
J.,
Yang
C.,
Zhang
W.,
What is the impact of human umbilical cord mesenchymal stem cell transplantation on clinical treatment?. Stem Cell Research & Therapy.
2020;
11
(1)
:
519
.
View Article Google Scholar -
Zhang
L.,
Li
Y.,
Guan
C.Y.,
Tian
S.,
Lv
X.D.,
Li
J.H.,
Therapeutic effect of human umbilical cord-derived mesenchymal stem cells on injured rat endometrium during its chronic phase. Stem Cell Research & Therapy.
2018;
9
(1)
:
36
.
View Article Google Scholar -
Fan
D.,
Zeng
M.,
Xia
Q.,
Wu
S.,
Ye
S.,
Rao
J.,
Efficacy and safety of umbilical cord mesenchymal stem cells in treatment of cesarean section skin scars: a randomized clinical trial. Stem Cell Research & Therapy.
2020;
11
(1)
:
244
.
View Article Google Scholar -
Kim
D.W.,
Staples
M.,
Shinozuka
K.,
Pantcheva
P.,
Kang
S.D.,
Borlongan
C.V.,
Wharton's jelly-derived mesenchymal stem cells: phenotypic characterization and optimizing their therapeutic potential for clinical applications. International Journal of Molecular Sciences.
2013;
14
(6)
:
11692-712
.
View Article Google Scholar -
Li
X.,
Bai
J.,
Ji
X.,
Li
R.,
Xuan
Y.,
Wang
Y.,
Comprehensive characterization of four different populations of human mesenchymal stem cells as regards their immune properties, proliferation and differentiation. International Journal of Molecular Medicine.
2014;
34
(3)
:
695-704
.
View Article Google Scholar -
Shang
Y.,
Guan
H.,
Zhou
F.,
Biological Characteristics of Umbilical Cord Mesenchymal Stem Cells and Its Therapeutic Potential for Hematological Disorders. Frontiers in Cell and Developmental Biology.
2021;
9
:
570179
.
View Article Google Scholar -
Nagamura-Inoue
T.,
He
H.,
Umbilical cord-derived mesenchymal stem cells: their advantages and potential clinical utility. World Journal of Stem Cells.
2014;
6
(2)
:
195-202
.
View Article Google Scholar -
Lindenmair
A.,
Hatlapatka
T.,
Kollwig
G.,
Hennerbichler
S.,
Gabriel
C.,
Wolbank
S.,
Mesenchymal stem or stromal cells from amnion and umbilical cord tissue and their potential for clinical applications. Cells.
2012;
1
(4)
:
1061-88
.
View Article Google Scholar -
Parolini
O.,
Alviano
F.,
Bagnara
G.P.,
Bilic
G.,
Bühring
H.J.,
Evangelista
M.,
Concise review: isolation and characterization of cells from human term placenta: outcome of the first international Workshop on Placenta Derived Stem Cells. Stem Cells (Dayton, Ohio).
2008;
26
(2)
:
300-11
.
View Article Google Scholar -
Ling
L.,
Feng
X.,
Wei
T.,
Wang
Y.,
Wang
Y.,
Wang
Z.,
Human amnion-derived mesenchymal stem cell (hAD-MSC) transplantation improves ovarian function in rats with premature ovarian insufficiency (POI) at least partly through a paracrine mechanism. Stem Cell Research & Therapy.
2019;
10
(1)
:
46
.
View Article Google Scholar -
Neuvians
T.P.,
Schams
D.,
Berisha
B.,
Pfaffl
M.W.,
Involvement of pro-inflammatory cytokines, mediators of inflammation, and basic fibroblast growth factor in prostaglandin F2α-induced luteolysis in bovine corpus luteum. Biology of Reproduction.
2004;
70
(2)
:
473-80
.
View Article Google Scholar -
Gan
L.,
Duan
H.,
Xu
Q.,
Tang
Y.Q.,
Li
J.J.,
Sun
F.Q.,
Human amniotic mesenchymal stromal cell transplantation improves endometrial regeneration in rodent models of intrauterine adhesions. Cytotherapy.
2017;
19
(5)
:
603-16
.
View Article Google Scholar -
Liu
H.,
Jiang
C.,
La
B.,
Cao
M.,
Ning
S.,
Zhou
J.,
Human amnion-derived mesenchymal stem cells improved the reproductive function of age-related diminished ovarian reserve in mice through Ampk/FoxO3a signaling pathway. Stem Cell Research & Therapy.
2021;
12
(1)
:
317
.
View Article Google Scholar -
Zhang
Q.,
Lai
D.,
Application of human amniotic epithelial cells in regenerative medicine: a systematic review. Stem Cell Research & Therapy.
2020;
11
(1)
:
439
.
View Article Google Scholar -
Liu
Q.W.,
Huang
Q.M.,
Wu
H.Y.,
Zuo
G.S.,
Gu
H.C.,
Deng
K.Y.,
Characteristics and therapeutic potential of human amnion-derived stem cells. International Journal of Molecular Sciences.
2021;
22
(2)
:
970
.
View Article Google Scholar -
Burton
G.J.,
Fowden
A.L.,
The placenta: a multifaceted, transient organ. Philosophical Transactions of the Royal Society B: Biological Sciences.
1663;
370
(1663)
:
20140066
.
View Article Google Scholar -
Torre
P. de la,
Pérez-Lorenzo
M.J.,
Flores
A.I.,
Human Placenta-Derived Mesenchymal Stromal Cells: A Review from Basic Research to Clinical Applications. Stromal Cells-Structure, Function, and Therapeutic Implications. IntechOpen. Stromal Cells Struct. Funct. Ther. Implic.
2019
.
View Article Google Scholar -
Lee
J.M.,
Jung
J.,
Lee
H.J.,
Jeong
S.J.,
Cho
K.J.,
Hwang
S.G.,
Comparison of immunomodulatory effects of placenta mesenchymal stem cells with bone marrow and adipose mesenchymal stem cells. International Immunopharmacology.
2012;
13
(2)
:
219-24
.
View Article Google Scholar -
Levy
J.A.,
Marchand
M.,
Iorio
L.,
Zribi
G.,
Zahalsky
M.P.,
Effects of stem cell treatment in human patients with Peyronie disease. The Journal of the American Osteopathic Association.
2015;
115
(10)
:
e8-13
.
-
Levy
J.A.,
Marchand
M.,
Iorio
L.,
Cassini
W.,
Zahalsky
M.P.,
Determining the feasibility of managing erectile dysfunction in humans with placental-derived stem cells. The Journal of the American Osteopathic Association.
2016;
116
(1)
:
e1-5
.
View Article Google Scholar -
Barak
S.,
Baker
H.G.,
Clinical management of male infertility. Endotext [Internet]. 2016 Feb 5.. 2016
.
View Article Google Scholar -
Kim
T.H.,
Choi
J.H.,
Jun
Y.,
Lim
S.M.,
Park
S.,
Paek
J.Y.,
3D-cultured human placenta-derived mesenchymal stem cell spheroids enhance ovary function by inducing folliculogenesis. Scientific Reports.
2018;
8
(1)
:
15313
.
View Article Google Scholar -
Camussi
G.,
Deregibus
M.C.,
Cantaluppi
V.,
Role of stem-cell-derived microvesicles in the paracrine action of stem cells. Biochemical Society Transactions.
2013;
41
(1)
:
283-7
.
View Article Google Scholar -
Zhang
Y.,
Liu
Y.,
Liu
H.,
Tang
W.H.,
Exosomes: biogenesis, biologic function and clinical potential. Cell & Bioscience.
2019;
9
(1)
:
19
.
View Article Google Scholar -
Álvarez-Viejo
M.,
Mesenchymal stem cells from different sources and their derived exosomes: A pre-clinical perspective. World Journal of Stem Cells.
2020;
12
(2)
:
100-9
.
View Article Google Scholar -
Javeed
N.,
Mukhopadhyay
D.,
Exosomes and their role in the micro-/macro-environment: a comprehensive review. Journal of Biomedical Research.
2017;
31
(5)
:
386-94
.
View Article Google Scholar -
Maia
J.,
Caja
S.,
Moraes
M.C. Strano,
Couto
N.,
Costa-Silva
B.,
Exosome-based cell-cell communication in the tumor microenvironment. Frontiers in Cell and Developmental Biology.
2018;
6
:
18
.
View Article Google Scholar -
Machtinger
R.,
Laurent
L.C.,
Baccarelli
A.A.,
Extracellular vesicles: roles in gamete maturation, fertilization and embryo implantation. Human Reproduction Update.
2016;
22
(2)
:
182-93
.
View Article Google Scholar -
Fortin
J.,
Boehm
U.,
Weinstein
M.B.,
Graff
J.M.,
Bernard
D.J.,
Follicle-stimulating hormone synthesis and fertility are intact in mice lacking SMAD3 DNA binding activity and SMAD2 in gonadotrope cells. The FASEB Journal.
2014;
28
(3)
:
1474-85
.
View Article Google Scholar -
Kharazi
U.,
Badalzadeh
R.,
A review on the stem cell therapy and an introduction to exosomes as a new tool in reproductive medicine. Reproductive Biology.
2020;
20
(4)
:
447-59
.
View Article Google Scholar -
Li
N.,
Zhao
L.,
Wei
Y.,
Ea
V.L.,
Nian
H.,
Wei
R.,
Recent advances of exosomes in immune-mediated eye diseases. Stem Cell Research & Therapy.
2019;
10
(1)
:
278
.
View Article Google Scholar -
Lee
J.K.,
Park
S.R.,
Jung
B.K.,
Jeon
Y.K.,
Lee
Y.S.,
Kim
M.K.,
Exosomes derived from mesenchymal stem cells suppress angiogenesis by down-regulating VEGF expression in breast cancer cells. PLoS One.
2013;
8
(12)
:
e84256
.
View Article Google Scholar -
Burden
H.P.,
Holmes
C.H.,
Persad
R.,
Whittington
K.,
Prostasomes - their effects on human male reproduction and fertility. Human Reproduction Update.
2006;
12
(3)
:
283-92
.
View Article Google Scholar -
Rubinstein
E.,
Ziyyat
A.,
Prenant
M.,
Wrobel
E.,
Wolf
J.P.,
Levy
S.,
Reduced fertility of female mice lacking CD81. Developmental Biology.
2006;
290
(2)
:
351-8
.
View Article Google Scholar -
Ohnami
N.,
Nakamura
A.,
Miyado
M.,
Sato
M.,
Kawano
N.,
Yoshida
K.,
CD81 and CD9 work independently as extracellular components upon fusion of sperm and oocyte. Biology Open.
2012;
1
(7)
:
640-7
.
View Article Google Scholar -
Hedlund
M.,
Stenqvist
A.C.,
Nagaeva
O.,
Kjellberg
L.,
Wulff
M.,
Baranov
V.,
Human placenta expresses and secretes NKG2D ligands via exosomes that down-modulate the cognate receptor expression: evidence for immunosuppressive function. Journal of Immunology (Baltimore, Md.: 1950).
2009;
183
(1)
:
340-51
.
View Article Google Scholar -
Abrahams
V.M.,
Straszewski-Chavez
S.L.,
Guller
S.,
Mor
G.,
First trimester trophoblast cells secrete Fas ligand which induces immune cell apoptosis. MHR: Basic science of reproductive medicine.
2004;
10
(1)
:
55-63
.
View Article Google Scholar -
Mincheva-Nilsson
L.,
Baranov
V.,
The role of placental exosomes in reproduction. American Journal of Reproductive Immunology (New York, N.Y.).
2010;
63
(6)
:
520-33
.
View Article Google Scholar -
Giunti
D.,
Marini
C.,
Parodi
B.,
Usai
C.,
Milanese
M.,
Bonanno
G.,
Role of miRNAs shuttled by mesenchymal stem cell-derived small extracellular vesicles in modulating neuroinflammation. Scientific Reports.
2021;
11
(1)
:
1740
.
View Article Google Scholar -
Mathieu
J.,
Ruohola-Baker
H.,
Regulation of Stem Cell Populations by microRNAs. In: Hime, G., Abud, H. (eds) Transcriptional and Translational Regulation of Stem Cells. Advances in Experimental Medicine and Biology, vol 786. Springer, Dordrecht 2013.
View Article Google Scholar -
Fu
X.,
He
Y.,
Wang
X.,
Peng
D.,
Chen
X.,
Li
X.,
Overexpression of miR-21 in stem cells improves ovarian structure and function in rats with chemotherapy-induced ovarian damage by targeting PDCD4 and PTEN to inhibit granulosa cell apoptosis. Stem Cell Research & Therapy.
2017;
8
(1)
:
187
.
View Article Google Scholar -
Eppig
J.J.,
Oocyte-Somatic Cell Interactions During Oocyte Growth and Maturation in the Mammal. In: Browder, L.W. (eds) Oogenesis. Developmental Biology, vol 1. Springer, Boston, MA 1985.
View Article Google Scholar -
Buscaglia
L.E.,
Li
Y.,
Apoptosis and the target genes of microRNA-21. Chinese Journal of Cancer.
2011;
30
(6)
:
371-80
.
View Article Google Scholar -
Zhang
J.,
Li
S.,
Li
L.,
Li
M.,
Guo
C.,
Yao
J.,
Exosome and exosomal microRNA: trafficking, sorting, and function. Genomics, Proteomics & Bioinformatics.
2015;
13
(1)
:
17-24
.
View Article Google Scholar -
Sun
B.,
Ma
Y.,
Wang
F.,
Hu
L.,
Sun
Y.,
miR-644-5p carried by bone mesenchymal stem cell-derived exosomes targets regulation of p53 to inhibit ovarian granulosa cell apoptosis. Stem Cell Research & Therapy.
2019;
10
(1)
:
360
.
View Article Google Scholar -
Yang
M.,
Lin
L.,
Sha
C.,
Li
T.,
Zhao
D.,
Wei
H.,
Bone marrow mesenchymal stem cell-derived exosomal miR-144-5p improves rat ovarian function after chemotherapy-induced ovarian failure by targeting PTEN. Laboratory Investigation.
2020;
100
(3)
:
342-52
.
View Article Google Scholar -
Sherbeny
M.F. El,
Laparoscopic intra-ovarian platelet rich plasma injection for ovarian rejuvenation: a new hope for infertile women. International Journal of Reproduction, Contraception, Obstetrics and Gynecology.
2020;
9
(10)
:
4320-3
.
View Article Google Scholar -
Su
J.,
Ding
L.,
Cheng
J.,
Yang
J.,
Li
X.,
Yan
G.,
Transplantation of adipose-derived stem cells combined with collagen scaffolds restores ovarian function in a rat model of premature ovarian insufficiency. Human Reproduction (Oxford, England).
2016;
31
(5)
:
1075-86
.
View Article Google Scholar -
Kadam
P.,
Ntemou
E.,
Baert
Y.,
Laere
S. Van,
Saen
D. Van,
Goossens
E.,
Co-transplantation of mesenchymal stem cells improves spermatogonial stem cell transplantation efficiency in mice. Stem Cell Research & Therapy.
2018;
9
(1)
:
317
.
View Article Google Scholar
Comments
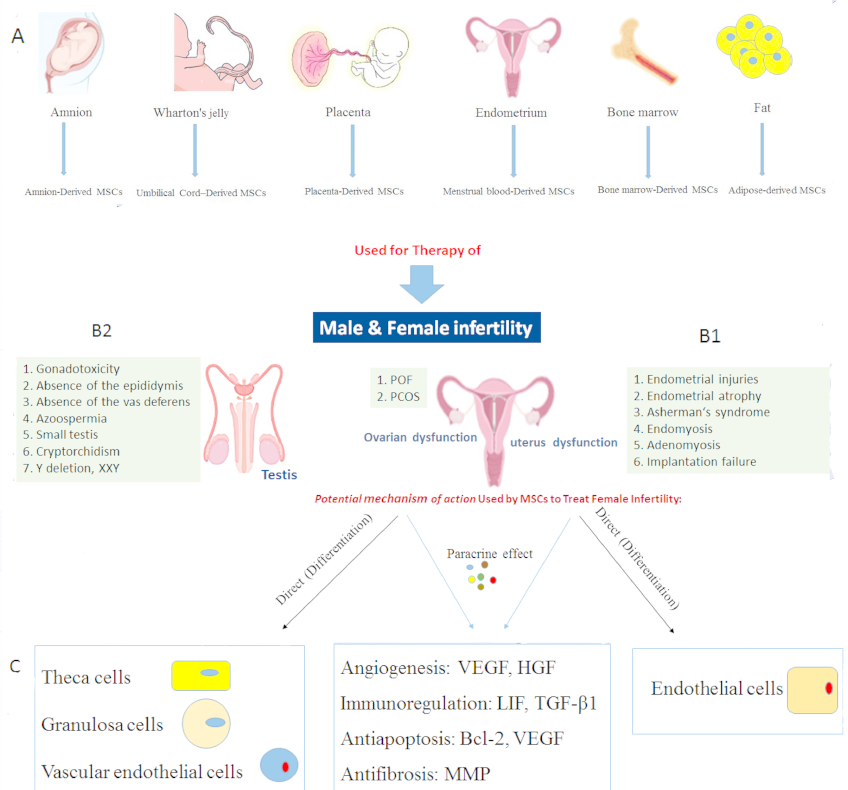
Article Details
Volume & Issue : Vol 10 No 6 (2023)
Page No.: 5744-5756
Published on: 2023-06-30
Citations
Copyrights & License

This work is licensed under a Creative Commons Attribution 4.0 International License.
Search Panel
Pubmed
Google Scholar
Pubmed
Google Scholar
Pubmed
Google Scholar
Pubmed
Google Scholar
Pubmed
Google Scholar
Pubmed
Search for this article in:
Google Scholar
Researchgate
- HTML viewed - 4933 times
- PDF downloaded - 1376 times
- XML downloaded - 107 times