Abstract
Introduction: Breast cancer has been one of the most frequently diagnosed cancers in women over the past decade. The presence of a breast cancer stem cell (BCSC) population within breast tumors significantly contributes to malignancy and drug resistance. Under the hypoxic conditions of breast tumors, BCSCs exhibit increased resistance to chemotherapy, complicating treatment and reducing its efficacy. Consequently, targeting BCSCs is considered a crucial strategy for breast cancer treatment. We hypothesize that the chemotherapy resistance of BCSCs is dependent on the upregulation of autophagy triggered by hypoxia. This study aims to investigate the relationship between autophagy and drug resistance of BCSCs in hypoxic conditions.
Methods: BCSCs were isolated from Vietnamese breast cancer cell line 1 (VNBRCA1) based on expression of CD44 and CD24. Cobalt (II) chloride (CoCl2) was used to simulate a hypoxic environment in monolayer cell cultures. The hypoxic status of BCSCs was confirmed by the upregulation of the HIF-1α gene. Autophagy activation was assessed through the expression of autophagy-related genes (LC3B, Beclin-1, AMPK, PI3K, AKT). Drug resistance was evaluated by measuring the expression of ABCC10 and assessing cell proliferation under cisplatin treatment.
Results: The study revealed that CoCl2 treatment led to high expression of HIF-1α and significant upregulation of LC3B in BCSCs, together with enhanced expression of ABCC10. Consequently, BCSCs exhibited marked resistance to cisplatin in the hypoxic environment. However, inhibition of autophagy using the autophagy inhibitor 3-methyladenine (3-MA) did not reduce cisplatin resistance in hypoxic conditions.
Conclusion: The findings indicate that in hypoxic conditions, BCSCs enhance both the autophagy process and drug resistance. However, the drug resistance of BCSCs in hypoxic conditions is not directly associated with the upregulation of autophagy. Therefore, targeting autophagy inhibition may not be an effective strategy to reduce drug resistance in hypoxia.
Introduction
According to the 2022 GLOBOCAN statistics, breast cancer is the second most common cancer, making up 11.5% of the 19,976,499 new cases, and ranks fourth in cancer-related deaths, accounting for 6.8% of the 9,743,832 fatalities globally. Furthermore, this data indicates that in Viet Nam, breast cancer represented the highest proportion of new cancer diagnoses in 2022, with the majority of patients being women. Primary prevention measures like reducing excess body weight, lowering alcohol consumption, increasing physical activity, and promoting breastfeeding can help reduce breast cancer incidence. In 2021, WHO launched the Global Breast Cancer Initiative to reduce mortality by 2.5% annually, aiming to save 2.5 million lives in 20 years through health promotion, timely diagnosis, and comprehensive management1.
Cancer stem cells (CSCs) play an important role in contributing to the heterogeneity of many kinds of cancer2. About 20 years ago, Al-Hajj et al. determined that a small breast cancer cell population strongly expressed CD44 and weakly expressed CD24, which was able to develop tumors in NOD/SCID mouse models3. Later, ALDH1 was also detected as a novel marker for breast cancer stem cell isolation, which is correlated with poor clinical outcomes in breast cancer patients4. Breast cancer stem cells are significant due to their role in metastasis and therapy resistance. Efforts are focused on characterizing them, understanding their pathways, and developing targeted therapies to eradicate them. New therapeutic approaches, including drug delivery, gene targeting, and immunotherapy, hold promise for effectively targeting breast cancer stem cells in cancer treatment5.
Cancer stem cells are usually located at the core of solid tumors, distant from the blood vessels, creating a hypoxic microenvironment (hypoxia). In comparison, while the level of oxygen pressure in normal tissue reaches over 40 mmHg, concentration in cancer tumors is frequently maintained between 0 and 20 mmHg. Transcription factors, which are responsible for adapting to hypoxic conditions, are known as hypoxia-induced factors (HIFs)6. We have reviewed the important effects of hypoxia as well as the roles of HIFs during tumor progression7. Particularly, HIF-1α and HIF-2α regulate cancer stem cells' adaptation to hypoxia and resistance to therapies8.
The term “autophagy” comes from a Greek word meaning “eating itself” and was first introduced in 1963 by a Belgian physiologist Christian de Duve. Mechanisms related to this biological process were studied and published by Yoshinori Ohsumi and his colleagues from the 1960s to the 1990s9, 10. This multi-step process includes autophagy induction, recognition, autophagosome formation, fusion with lysosome, and autophagolysosome destruction11. Each step involves the regulation of many genes called autophagy-related genes (ATGs). At least 40 ATGs have been discovered and 20 of them play key roles in the autophagy of eukaryotic cells12. In cancer cells, autophagy acts not only as a "friend" since it stimulates cancer cells to proliferate, but also as a "foe" due to its inhibitory impacts on cancer cell survival13. In the case of tumor suppression, autophagy protects cancer cells by preventing the accumulation of DNA-damaging reactive oxygen species (ROS) and by removing damaged proteins and organelles to maintain homeostasis14, 15. Conversely, autophagy activation also aids tumor cell survival by regulating various mediators16, 17. Overall, the process of autophagy in cancer cells is highly complex and not yet fully understood.
Hypoxia has been shown to induce autophagy in some types of cancer stem cells18, 19, which has led to studies on signaling pathways related to autophagy in hypoxic cancer stem cells. In this study, we use cobalt (II) chloride (CoCl2) to mimic hypoxic conditions in breast cancer stem-like cell populations to investigate changes in the expression of genes related to autophagy induction and regulation. Additionally, we examine changes in drug resistance gene expression ABCC10 and cisplatin resistance in breast cancer stem-like cells in a hypoxia-mimic model. Based on these results, we draw preliminary conclusions about the molecular relationship between autophagy and drug resistance in hypoxic breast cancer stem-like cells.
Methods
Cell Culture
VNBRCA1 stands for the Vietnamese breast cancer cell line no. 1, which was isolated and characterized from Vietnamese breast cancer patients and was described and used by our laboratory in previous studies20. Cancer cells were cultured in DMEM (Lonza, Switzerland) supplemented with 10% FBS (Thermo Fisher Scientific, USA) and 1% antimycotic-antibiotic (HyClone, USA) for enrichment in a T-25 flask (SPL Life Science, Korea Republic). All flasks were incubated at 37°C and 5% CO2, and the medium was freshly changed after 48 to 72 hours. After reaching 80-90% confluency, cells were harvested by Deattachment reagent (Regenmedlab, Viet Nam) to perform subculture.
Flow cytometry assay for CD44highCD24low population
Cells were harvested by Deattachment reagent as described above. Approximately 1×107 VNBRCA1 cells were stained with 10 µL of anti-CD44 (BD Biosciences, USA) and 10 µL of anti-CD24 (Sigma-Aldrich, USA) in phosphate-buffered saline (supplemented with 2% BSA) at 4°C in the dark for 20 minutes. Then, all cells were sorted based on fluorescent-bound antigen signals using the BD FACSMelody Cell Sorter (BD Biosciences, USA). The cell population that is strongly positive for CD44 and negatively or dimly expressed CD24 is considered breast cancer stem cells (BCSCs). They are marked as BCSCs hereafter in this article. BCSCs were cultured in M171 medium (Thermo Fisher Scientific, USA) supplemented with 1% MEGS (Thermo Fisher Scientific, USA) at 37°C and 5% CO2.
Cobalt (II) Chloride Cytotoxicity Assay
Cytotoxicity was determined using the AlamarBlue assay21. BCSCs were seeded at a density of 3,000 cells per well in a 96-well plate (SPL Life Science, Korea Republic). Each well was filled with 100 µL of M171. After 24 hours of incubation, the medium was withdrawn and replaced with new medium containing CoCl₂ at different concentrations. Medium without CoCl₂ was used as a negative control. The percentage of cell viability was calculated based on the following formula:
Cisplatin Cytotoxicity Assay
Breast cancer stem-like cells were seeded at a density of 3,000 cells per well in a 96-well plate. They were then pretreated with different concentrations of CoCl₂ for various periods of time. Subsequently, the M171 medium was withdrawn and replaced with new medium containing cisplatin (Sigma-Aldrich, USA) at concentrations of 50, 100, 150, 200, and 250 µM, respectively. BCSCs were incubated at 37°C for the next 24 hours, and cell viability was assessed via the AlamarBlue assay as described above.
Gene Expression Analysis
RNA isolation was performed using the easy-BLUETM Total RNA Extraction Kit (iNtRON Biotechnology, South Korea). The expression levels of target genes were determined by real-time quantitative RT-PCR (reverse transcriptase polymerase chain reaction) with specific primers (listed in Table 1). All primers were purchased from PHUSA Biochem Co., Viet Nam. RNA samples were divided into qPCR tubes (Thermo Fisher Scientific, USA) with appropriate amounts and supplemented with the necessary components for a standard PCR reaction, following the instructions in the Luna Universal One-Step RT-qPCR Kit (New England Biolabs, USA). Thermal cycling was performed using the Replex system (Eppendorf, USA). Gene expression levels were semi-quantitated using the Livak formula, with β-actin used for normalization.
Genes | Primer sequence (5'-3') | Referrence |
β -actin F | CTGGAACGGTGAAGGTGACA | 22 |
β -actin R | AAGGGACTTCCTGTAACAATGCA | |
HIF-1 α F | TGGTGACATGATTTACATTTCTGA | 23 |
HIF-1 α R | AAGGCCATTTCTGTGTGTAAGC | |
LC3B F | TGTCCGACTTATTCGAGAGCAGCA | 24 |
LC3B R | TTCACCAACAGGAAGAAGGCCTGA | |
Beclin-1 F | ATGCAGGTGAGCTTCGTGTG | 25 |
Beclin-1 R | CTGGGCTGTGGTAAGTAATGGA | |
ABCC10 F | CGGGTTAAGCTTGTGACAGAGC | 26 |
ABCC10 R | AACACCTTGGTGGCAGTGAGCT | |
AMPK F | CAGGCATATGGTGGTCCATAGAG | 27 |
AMPK R | TCATGGGATCCACCTGCAGC | |
PI3K F | TGTGTGGGACTTATTGAGGTG | 28 |
PI3K R | ACACAGTATCCAGCACATGAAC | |
AKT F | TCTATGGCGCTGAGATTGTG | 29 |
AKT R | CTTAATGTGCCCGTCCTTGT |
Statistical Analysis
All assays were performed in triplicate. Raw data were collected using Microsoft Excel 365 and analyzed with GraphPad Prism 9.0 software. All data are displayed as mean ± standard error of the mean (SEM). Differences between treatments were examined using the one-way ANOVA method, with a p-value < 0.05 considered statistically significant.
Results
Establishing model of hypoxic BCSCs using CoCl2
After sorting, the BCSC population predominantly exhibits a CD44highCD24low phenotype (over 96%) and shows an approximately 1.5-fold increase in ALDH1 gene expression compared to the pre-sorting population (Figure 1A). However, this increase in ALDH1 expression is not statistically significant (p-value = 0.20) (Figure 1B). This population was used for further analysis.
In the first assay to evaluate the cytotoxicity of CoCl2, CoCl2 displayed high toxicity in BCSCs when used in high concentrations. Indeed, when compared to cells not treated with CoCl2, the survival rate of BCSCs markedly decreases in a concentration- and time-dependent manner when exposed to CoCl2 concentrations ranging from 100 to 800 μM for 24 to 72 hours (Figure 1C). In contrast, BCSCs exposed to CoCl2 concentrations below 100 μM for 24 hours show a generally reduced survival rate, but it is not significantly different from the negative control (Figure 1D).
At low concentrations of CoCl2 (25 to 100 μM), BCSCs demonstrated a significant rise in HIF-1α gene expression. Notably, exposure to 100 μM CoCl2 results in the most significant increase, with a 4.54-fold elevation in HIF-1α gene expression compared to cells not treated with CoCl2 (Figure 1E).
Autophagy is stimulated under hypoxic condition
The hypoxic environment caused by adding CoCl2 strongly induced autophagy in BCSCs. In fact, in the presence of CoCl2, LC3B, a central marker of autophagy induction, exhibits increased gene expression in a concentration-dependent manner. As CoCl2 concentration rises, LC3B gene expression increases from a 1.45 to a 4.09-fold change compared to untreated cells. The expression of the Beclin-1 gene, another autophagy marker, also increases at CoCl2 concentrations of 50, 75, and 100 µM (1.49, 2.28, and 1.64-fold change, respectively), but it shows no significant change at 25 µM (p > 0.05). Overall, both genes display increased expression with higher CoCl2 concentrations, although these changes are not statistically significant (p > 0.05) (Figure 2A). In general, genes involved in autophagy regulation exhibit elevated expression levels when exposed to various CoCl2 concentrations (Figure 2B). The AMPK gene shows the greatest expression increase at 50 µM CoCl2 (3.14-fold change), while PI3K and AKT genes reach their peak expression at 75 µM CoCl2 (2.69 and 2.14-fold change, respectively). After reaching peak expression, the levels of these genes gradually decline with further increases in CoCl2 concentration.
The inhibition of autophagy did not reduce the drug resistance of BCSCs
The potential for drug resistance in BCSCs was assessed using a cytotoxicity assay with the anti-tumor drug cisplatin. The drug resistance of hypoxic BCSCs to cisplatin was evaluated either in the presence or absence of the autophagy inhibitor 3-MA in the culture medium. In this assay, BCSCs were first induced to become hypoxic by culturing them in a medium containing CoCl2 (ranging from 25 to 100 μM) for 24 hours. Subsequently, these hypoxic BCSCs were cultured in a fresh medium containing 60 μM cisplatin for the next 24 hours.
The AlamarBlue assay results indicated that the viability of hypoxic BCSCs increased with the concentration of cobalt (II) chloride, although the increase was not significant. Concurrent treatment with 60 μM cisplatin and 5 mM autophagy inhibitor 3-MA also enhanced the viability of the BCSCs population in relation to the concentration of CoCl2.
The results showed that, whether treated with or without 3-MA, the cell viability of BCSCs remained similar and peaked at 75 μM CoCl2 (Figure 3).
Hypoxia enhanced the expression of ABCC10
Treatment with CoCl2 results in an upregulation of the drug resistance gene ABCC10. The most significant increase in expression, observed as a 9.17-fold change, occurs at a concentration of 75 μM CoCl2 compared to breast cancer cells not exposed to CoCl2 (see Figure 4).
Discussion
The Cluster of Differentiation 44 (CD44) is a complex transmembrane adhesion glycoprotein involved in numerous signaling pathways significant in carcinogenesis and tumor progression30. Meanwhile, Cluster of Differentiation 24 (CD24) serves as a crucial biomarker, indicating poor prognoses like increased invasion, metastasis, and reduced survival in cancer patients31. The enzyme Aldehyde dehydrogenase (ALDH1) catalyzes the conversion of intracellular aldehydes to carboxylic acids and is expressed highly in a small subset of breast tumor cells capable of tumor generation in implanted mice32. Breast cancer cells displaying the CD44+CD24–/lowALDH1high phenotype are considered cancer stem cells due to their association with poorer outcomes in breast cancer patients33. In this study, breast cancer cells isolated from Vietnamese patients were cultured in DMEM with 10% FBS to expand their population. Flow cytometry sorting was then used to purify cells with high CD44 expression and low CD24 expression. As such, CD44highCD24low breast cancer cells exhibiting elevated ALDH1 and EpCAM can be classified as breast cancer stem-like cells (BCSCs).
Cobalt (II) chloride (CoCl2) has been used previously to modulate HIF expressions in various cancer34, 35, 36 and non-cancer cell lines37, 38. Past studies commonly used CoCl2 concentrations over 100 μM; however, our research demonstrated that BCSC proliferation was significantly inhibited at concentrations above 100 μM for extended durations (24 to 72 hours). Consequently, we opted for lower concentrations of CoCl2, under 100 μM, administered for shorter times (4 to 24 hours). The AlamarBlue assay revealed reduced BCSC proliferation, with no significant difference compared to cells cultured without CoCl2. Similarly, a Thai study reported comparable outcomes using CoCl2 concentrations of 50 or 100 μM39. To prevent extensive cell death affecting other evaluations, we employed CoCl2 concentrations ranging from 25 to 100 μM in subsequent experiments.
Autophagy under controlled hypoxia promotes cancer cell survival; however, under severe hypoxic stress, it can lead to cell death40. In normoxic conditions, hypoxia-inducing factors (HIFs) are deactivated as prolyl hydroxylase enzymes hydroxylate them using oxygen. In hypoxia, HIFs stabilize and translocate to the nucleus, activating oncogenes41. Among HIF isoforms, HIF-1α is the most studied due to its mRNA levels increasing with the severity of hypoxia in many cancers42, 43, 44. Thus, HIF-1α can serve as a biomarker for evaluating tumor hypoxia levels. Using RT-qPCR, we found that CD44highCD24low breast cancer cells exhibited increased HIF-1α expression correlating with rising CoCl2 concentrations. Various mechanisms involving cobalt ions (Co2+) enhancing HIF-1α expression have been suggested, generally affecting cofactors, inhibiting PHDs, and stabilizing HIF-1α45. Higher CoCl2 concentrations in experimental models reflect a more pronounced hypoxic state in cancer tumors.
Beclin-1 is essential for signaling pathways activating autophagy and autophagosome formation, requiring interaction with hVsp34, while LC3B, a lipidated form of microtubule-associated protein light-chain 3, is a reliable autophagy marker, increasing in response to stressors46. Our study found that Beclin-1 and LC3B gene expressions increased with rising CoCl2 concentrations, indicating strong autophagy induction by this agent, similar to results in other cell lines47. The observed increase in HIF-1α expression suggests a link between autophagy and hypoxic response in BCSCs. According to Chen et al., CoCl2 exposure increased HIF-1α and its downstream protein BNIP3, involved in autophagy induction through interaction with Beclin-1 and LC347. The AMPK/PI3K/AKT pathway is implicated in autophagy across mammalian cell lines, typically with AMPK activation and increased expression, while PI3K and AKT expressions decrease under autophagy-inducing agents48, 49, 50. However, our results showed all three genes increased expression in BCSCs exposed to 25-75 μM CoCl2, decreasing at 100 μM. Prior research also indicated mTOR, downstream of PI3K and AKT, plays a key role in autophagy regulation, with reduced PI3K and AKT expressions potentially inhibiting mTOR and initiating autophagy51. AMPK activation relates to numerous autophagy process steps, given its involvement in several autophagy factors52. Variances in gene expressions within BCSCs suggest autophagy regulation through alternate signaling pathways, necessitating further investigation into AMPK/PI3K/AKT and autophagy relationships.
Autophagy links to drug resistance in different cancer cell lines. Experiments with cisplatin on CD44highCD24low BCSCs, treated with CoCl2 to simulate hypoxia and stimulate autophagy, showed increased cell survival correlating with CoCl2 concentrations. This survival increase aligned with changes in autophagy-related gene expressions and ABCC10, a gene associated with drug resistance in breast cancer stem cells26. We earlier posited that autophagy acts as a "double-edged sword" in drug resistance among cancer stem cells, potentially enhancing sensitivity or decreasing responsiveness to chemotherapy drugs based on the signaling pathway involved53. Thus, targeting autophagy may offer a strategy to combat drug resistance in cancer cells. Numerous studies revealed that inhibiting autophagy through chemicals or genetic alterations enhances apoptosis in chemotherapy-resistant cell lines54, 55, 56, 57. We hypothesize that autophagy suppression increases sensitivity to cisplatin in CD44highCD24low BCSCs. Using 5 mM 3-MA, an autophagy inhibitor, we observed significant LC3B expression reduction compared to untreated cells (data not shown). Nevertheless, the response to cisplatin remained unchanged under 3-MA influence, suggesting a weaker linkage between autophagy and cisplatin response in our cell line compared to others. Hence, more specific methods are required to evaluate signaling pathways linking these properties in Vietnamese breast cancer stem-like cells.
Conclusions
BCSCs exhibit increased expression of the HIF-1α gene when exposed to higher concentrations of cobalt (II) chloride (CoCl2), which also leads to the upregulation of genes involved in the induction (Beclin-1, LC3B) and regulation (AMPK, PI3K, AKT) of autophagy. Under hypoxia-mimicking conditions, BCSCs show increased resistance to cisplatin. However, inhibiting autophagy with the inhibitor 3-MA does not reduce the drug resistance of hypoxic BCSCs. Furthermore, under these conditions, an increase in the expression of the drug resistance gene ABCC10 is observed in response to CoCl2 levels. These findings suggest that the drug resistance of BCSCs under hypoxia is related to both autophagy upregulation and ABCC10 expression. Therefore, addressing drug resistance in BCSCs should consider both autophagy and ABCC10 pathways.
Abbreviations
3-MA: 3-Methyladenine, ABCC10: ATP-Binding Cassette Subfamily C Member 10, AKT: Protein Kinase B, AMPK: AMP-Activated Protein Kinase, ANOVA: Analysis of Variance, BCSC: Breast Cancer Stem Cell, CoCl2: Cobalt (II) Chloride, CSC: Cancer Stem Cell, DMEM: Dulbecco's Modified Eagle Medium, FBS: Fetal Bovine Serum, HIF: Hypoxia-Induced Factor, HIF-1α: Hypoxia-Induced Factor 1 alpha, LC3B: Microtubule-Associated Proteins 1A/1B Light Chain 3B, mRNA: Messenger Ribonucleic Acid, PI3K: Phosphoinositide 3-Kinase, RT-qPCR: Real-Time Quantitative Polymerase Chain Reaction, SEM: Standard Error of the Mean, VNBRCA1: Vietnamese Breast Cancer Cell Line No. 1
Acknowledgments
We especially thank Center of Research and Development – Stem Cell Institute for creating favorable conditions for us to carry out this project.
Author’s contributions
Bui Dinh Khan and Tran Ngo The Nhan equally contributed to developing the research methodology, conducting the experimental work, and collecting the data. Bui Dinh Khan took primary responsibility for data analysis and manuscript content. Pham Van Phuc suggested the layout, discussed the results, revised entire manuscript, and supervised the study. All authors contributed significantly to this work and have read and approved the final version of the manuscript.
Funding
This paper is funded by University of Science, VNU-HCM under grant number T2021-62.
Availability of data and materials
Data and materials used and/or analyzed during the current study are available from the corresponding author on reasonable request.
Ethics approval and consent to participate
Not applicable.
Consent for publication
Not applicable.
Competing interests
The authors declare that they have no competing interests.
References
-
Bray
F.,
Laversanne
M.,
Sung
H.,
Ferlay
J.,
Siegel
R.L.,
Soerjomataram
I.,
Global cancer statistics 2022: GLOBOCAN estimates of incidence and mortality worldwide for 36 cancers in 185 countries. CA: a Cancer Journal for Clinicians.
2024;
74
(3)
:
229-63
.
View Article PubMed Google Scholar -
Beck
B.,
Blanpain
C.,
Unravelling cancer stem cell potential. Nature Reviews. Cancer.
2013;
13
(10)
:
727-38
.
View Article PubMed Google Scholar -
Al-Hajj
M.,
Wicha
M.S.,
Benito-Hernandez
A.,
Morrison
S.J.,
Clarke
M.F.,
Prospective identification of tumorigenic breast cancer cells. Proceedings of the National Academy of Sciences of the United States of America.
2003;
100
(7)
:
3983-8
.
View Article PubMed Google Scholar -
Ginestier
C.,
Hur
M.H.,
Charafe-Jauffret
E.,
Monville
F.,
Dutcher
J.,
Brown
M.,
ALDH1 is a marker of normal and malignant human mammary stem cells and a predictor of poor clinical outcome. Cell Stem Cell.
2007;
1
(5)
:
555-67
.
View Article PubMed Google Scholar -
Scioli
M.G.,
Storti
G.,
D'Amico
F.,
Gentile
P.,
Fabbri
G.,
Cervelli
V.,
The role of breast cancer stem cells as a prognostic marker and a target to improve the efficacy of breast cancer therapy. Cancers (Basel).
2019;
11
(7)
:
1021
.
View Article PubMed Google Scholar -
Barth
D.A.,
Prinz
F.,
Teppan
J.,
Jonas
K.,
Klec
C.,
Pichler
M.,
Long-Noncoding RNA (lncRNA) in the regulation of hypoxia-inducible factor (HIF) in Cancer. Non-Coding RNA.
2020;
6
(3)
:
27
.
View Article PubMed Google Scholar -
Khan
B.D.,
Chau
H.N.,
Nhi
N.T.,
Roles of hypoxia in tumor progression and novel strategies for cancer treatment. Biomedical Research and Therapy.
2022;
9
(10)
:
5361-74
.
View Article Google Scholar -
Tong
W.W.,
Tong
G.H.,
Liu
Y.,
Cancer stem cells and hypoxia-inducible factors (Review). International Journal of Oncology.
2018;
53
(2)
:
469-76
.
View Article PubMed Google Scholar -
Ashrafizadeh
M.,
Ahmadi
Z.,
Mohammadinejad
R.,
Kaviyani
N.,
Tavakol
S.,
Monoterpenes modulating autophagy: A review study. Basic & Clinical Pharmacology & Toxicology.
2020;
126
(1)
:
9-20
.
View Article PubMed Google Scholar -
Mizushima
N.,
A brief history of autophagy from cell biology to physiology and disease. Nature Cell Biology.
2018;
20
(5)
:
521-7
.
View Article PubMed Google Scholar -
He
C.,
Klionsky
D.J.,
Regulation mechanisms and signaling pathways of autophagy. Annual Review of Genetics.
2009;
43
(1)
:
67-93
.
View Article PubMed Google Scholar -
Sakamoto
H.,
Nakada-Tsukui
K.,
Besteiro
S.,
The Autophagy Machinery in Human-Parasitic Protists; Diverse Functions for Universally Conserved Proteins. Cells.
2021;
10
(5)
:
1258
.
View Article PubMed Google Scholar -
Yun
C.W.,
Jeon
J.,
Go
G.,
Lee
J.H.,
Lee
S.H.,
The dual role of autophagy in cancer development and a therapeutic strategy for cancer by targeting autophagy. International Journal of Molecular Sciences.
2020;
22
(1)
:
179
.
View Article PubMed Google Scholar -
Guo
J.Y.,
Xia
B.,
White
E.,
Autophagy-mediated tumor promotion. Cell.
2013;
155
(6)
:
1216-9
.
View Article PubMed Google Scholar -
Bischof
J.,
Westhoff
M.A.,
Wagner
J.E.,
Halatsch
M.E.,
Trentmann
S.,
Knippschild
U.,
Cancer stem cells: the potential role of autophagy, proteolysis, and cathepsins in glioblastoma stem cells. Tumour Biology.
2017;
39
(3)
:
1010428317692227
.
View Article PubMed Google Scholar -
Hu
Y.L.,
DeLay
M.,
Jahangiri
A.,
Molinaro
A.M.,
Rose
S.D.,
Carbonell
W.S.,
Hypoxia-induced autophagy promotes tumor cell survival and adaptation to antiangiogenic treatment in glioblastoma. Cancer Research.
2012;
72
(7)
:
1773-83
.
View Article PubMed Google Scholar -
Gao
L.,
Dou
Z.C.,
Ren
W.H.,
Li
S.M.,
Liang
X.,
Zhi
K.Q.,
CircCDR1as upregulates autophagy under hypoxia to promote tumor cell survival via AKT/ERK/mTOR signaling pathways in oral squamous cell carcinomas. Cell Death & Disease.
2019;
10
(10)
:
745
.
View Article PubMed Google Scholar -
Samanta
D.,
Gilkes
D.M.,
Chaturvedi
P.,
Xiang
L.,
Semenza
G.L.,
Hypoxia-inducible factors are required for chemotherapy resistance of breast cancer stem cells. Proceedings of the National Academy of Sciences of the United States of America.
2014;
111
(50)
:
5429-38
.
View Article PubMed Google Scholar -
Qureshi-Baig
K.,
Kuhn
D.,
Viry
E.,
Pozdeev
V.I.,
Schmitz
M.,
Rodriguez
F.,
Hypoxia-induced autophagy drives colorectal cancer initiation and progression by activating the PRKC/PKC-EZR (ezrin) pathway. Autophagy.
2020;
16
(8)
:
1436-52
.
View Article PubMed Google Scholar -
Phuc
P. Van,
Isolation and characterization of breast cancer stem cells from malignant tumours in Vietnamese women. Journal of Cell and Animal Biology.
2010;
4
(12)
:
163-9
.
View Article Google Scholar -
Kumar
P.,
Nagarajan
A.,
Uchil
P.D.,
Analysis of cell viability by the alamarBlue assay. Cold Spring Harbor Protocols, 2018. 2018(6): p. pdb. prot095489. 2018
.
View Article Google Scholar -
Wu
S.,
Sun
C.,
Tian
D.,
Li
Y.,
Gao
X.,
He
S.,
Expression and clinical significances of Beclin1, LC3 and mTOR in colorectal cancer. International Journal of Clinical and Experimental Pathology.
2015;
8
(4)
:
3882-91
.
PubMed Google Scholar -
Li
J.Z.,
Wang
Z.L.,
Xu
W.H.,
Li
Q.,
Gao
L.,
Wang
Z.M.,
MicroRNA-495 regulates migration and invasion in prostate cancer cells via targeting Akt and mTOR signaling. Cancer Investigation.
2016;
34
(4)
:
181-8
.
View Article PubMed Google Scholar -
Akrami
H.,
Mahmoodi
F.,
Havasi
S.,
Sharifi
A.,
PlGF knockdown inhibited tumor survival and migration in gastric cancer cell via PI3K/Akt and p38MAPK pathways. Cell Biochemistry and Function.
2016;
34
(3)
:
173-80
.
View Article PubMed Google Scholar -
Wang
J.,
Ni
Z.,
Duan
Z.,
Wang
G.,
Li
F.,
Altered expression of hypoxia-inducible factor-1α (HIF-1α) and its regulatory genes in gastric cancer tissues. PLoS One.
2014;
9
(6)
:
e99835
.
View Article PubMed Google Scholar -
Li
G.,
Shan
C.,
Liu
L.,
Zhou
T.,
Zhou
J.,
Hu
X.,
Tanshinone IIA inhibits HIF-1α and VEGF expression in breast cancer cells via mTOR/p70S6K/RPS6/4E-BP1 signaling pathway. PLoS One.
2015;
10
(2)
:
e0117440
.
View Article PubMed Google Scholar -
Liu
Q.,
Fang
Q.,
Ji
S.,
Han
Z.,
Cheng
W.,
Zhang
H.,
Resveratrol-mediated apoptosis in renal cell carcinoma via the p53/AMP‑activated protein kinase/mammalian target of rapamycin autophagy signaling pathway. Molecular Medicine Reports.
2018;
17
(1)
:
502-8
.
PubMed Google Scholar -
Kohjima
M.,
Higuchi
N.,
Kato
M.,
Kotoh
K.,
Yoshimoto
T.,
Fujino
T.,
SREBP-1c, regulated by the insulin and AMPK signaling pathways, plays a role in nonalcoholic fatty liver disease. International Journal of Molecular Medicine.
2008;
21
(4)
:
507-11
.
View Article PubMed Google Scholar -
Sobczak
M.,
Strachowska
M.,
Gronkowska
K.,
Robaszkiewicz
A.,
Activation of ABCC Genes by Cisplatin Depends on the CoREST Occurrence at Their Promoters in A549 and MDA-MB-231 Cell Lines. Cancers (Basel).
2022;
14
(4)
:
894
.
View Article PubMed Google Scholar -
Xu
H.,
Niu
M.,
Yuan
X.,
Wu
K.,
Liu
A.,
CD44 as a tumor biomarker and therapeutic target. Experimental Hematology & Oncology.
2020;
9
(1)
:
36
.
View Article PubMed Google Scholar -
Fang
X.,
Zheng
P.,
Tang
J.,
Liu
Y.,
CD24: from A to Z. Cellular & Molecular Immunology.
2010;
7
(2)
:
100-3
.
View Article PubMed Google Scholar -
Douville
J.,
Beaulieu
R.,
Balicki
D.,
ALDH1 as a functional marker of cancer stem and progenitor cells. Stem Cells and Development.
2009;
18
(1)
:
17-25
.
View Article PubMed Google Scholar -
Ricardo
S.,
Vieira
A.F.,
Gerhard
R.,
Leitão
D.,
Pinto
R.,
Cameselle-Teijeiro
J.F.,
Breast cancer stem cell markers CD44, CD24 and ALDH1: expression distribution within intrinsic molecular subtype. Journal of Clinical Pathology.
2011;
64
(11)
:
937-46
.
View Article PubMed Google Scholar -
Mikeladze
M.A.,
Dutysheva
E.A.,
Kartsev
V.G.,
Margulis
B.A.,
Guzhova
I.V.,
Lazarev
V.F.,
Disruption of the complex between GAPDH and Hsp70 sensitizes C6 glioblastoma cells to hypoxic stress. International Journal of Molecular Sciences.
2021;
22
(4)
:
1520
.
View Article PubMed Google Scholar -
Tang
Y.,
Jin
Y.H.,
Li
H.L.,
Xin
H.,
Chen
J.D.,
Li
X.Y.,
PBRM1 deficiency oncogenic addiction is associated with activated AKT-mTOR signalling and aerobic glycolysis in clear cell renal cell carcinoma cells. Journal of Cellular and Molecular Medicine.
2022;
26
(14)
:
3837-49
.
View Article PubMed Google Scholar -
Yang
L.,
Zeng
L.,
Wang
Z.,
Hu
X.,
Xiong
H.,
Zhang
T.,
Differentiated embryo chondrocyte 1, induced by hypoxia-inducible factor 1α, promotes cell migration in oral squamous cell carcinoma cell lines. Oral Surgery, Oral Medicine, Oral Pathology and Oral Radiology.
2022;
133
(2)
:
199-206
.
View Article PubMed Google Scholar -
Nakuluri
K.,
Mukhi
D.,
Mungamuri
S.K.,
Pasupulati
A.K.,
Stabilization of hypoxia-inducible factor 1α by cobalt chloride impairs podocyte morphology and slit-diaphragm function. Journal of Cellular Biochemistry.
2019;
120
(5)
:
7667-78
.
View Article PubMed Google Scholar -
Zhang
C.,
Chen
M.,
Tao
Q.,
Chi
Z.,
Cobalt chloride-stimulated hypoxia promotes the proliferation of cholesteatoma keratinocytes via the PI3K/Akt signaling pathway. International Journal of Medical Sciences.
2021;
18
(15)
:
3403-11
.
View Article PubMed Google Scholar -
Jampasri
S.,
Reabroi
S.,
Tungmunnithum
D.,
Parichatikanond
W.,
Pinthong
D.,
Plumbagin Suppresses Breast Cancer Progression by Downregulating HIF-1α Expression via a PI3K/Akt/mTOR Independent Pathway under Hypoxic Condition. Molecules (Basel, Switzerland).
2022;
27
(17)
:
5716
.
View Article PubMed Google Scholar -
Mazure
N.M.,
Pouysségur
J.,
Hypoxia-induced autophagy: cell death or cell survival?. Current Opinion in Cell Biology.
2010;
22
(2)
:
177-80
.
View Article PubMed Google Scholar -
Pezzuto
A.,
Carico
E.,
Role of HIF-1 in cancer progression: novel insights. A review. Current Molecular Medicine.
2018;
18
(6)
:
343-51
.
View Article PubMed Google Scholar -
Yoshikawa
T.,
Tsuburaya
A.,
Miyagi
Y.,
Sekiguchi
H.,
Kimura
M.,
Cho
H.,
Up-regulation of hypoxia-inducible factor-1 alpha and VEGF mRNAs in peritoneal dissemination of patients with gastric cancer. Anticancer Research.
2006;
26
:
3849-53
.
PubMed Google Scholar -
Qin
J.,
Liu
Y.,
Lu
Y.,
Liu
M.,
Li
M.,
Li
J.,
Hypoxia-inducible factor 1 alpha promotes cancer stem cells-like properties in human ovarian cancer cells by upregulating SIRT1 expression. Scientific Reports.
2017;
7
(1)
:
10592
.
View Article PubMed Google Scholar -
Maxwell
P.J.,
Gallagher
R.,
Seaton
A.,
Wilson
C.,
Scullin
P.,
Pettigrew
J.,
HIF-1 and NF-kappaB-mediated upregulation of CXCR1 and CXCR2 expression promotes cell survival in hypoxic prostate cancer cells. Oncogene.
2007;
26
(52)
:
7333-45
.
View Article PubMed Google Scholar -
Muñoz-Sánchez
J.,
Chánez-Cárdenas
M.E.,
The use of cobalt chloride as a chemical hypoxia model. Journal of Applied Toxicology.
2019;
39
(4)
:
556-70
.
View Article PubMed Google Scholar -
Miracco
C.,
Cevenini
G.,
Franchi
A.,
Luzi
P.,
Cosci
E.,
Mourmouras
V.,
Beclin 1 and LC3 autophagic gene expression in cutaneous melanocytic lesions. Human Pathology.
2010;
41
(4)
:
503-12
.
View Article PubMed Google Scholar -
Chen
R.,
Effects of cobalt chloride, a hypoxia-mimetic agent, on autophagy and atrophy in skeletal C2C12 myotubes. ioMed research international.
2017;
2017
:
7097580
.
View Article PubMed Google Scholar -
Lin
Y.,
Kuang
W.,
Wu
B.,
Xie
C.,
Liu
C.,
Tu
Z.,
IL-12 induces autophagy in human breast cancer cells through AMPK and the PI3K/Akt pathway. Molecular Medicine Reports.
2017;
16
(4)
:
4113-8
.
View Article PubMed Google Scholar -
Yin
H.,
Zuo
Z.,
Yang
Z.,
Guo
H.,
Fang
J.,
Cui
H.,
Nickel induces autophagy via PI3K/AKT/mTOR and AMPK pathways in mouse kidney. Ecotoxicology and Environmental Safety.
2021;
223
:
112583
.
View Article PubMed Google Scholar -
Hu
S.,
Yin
J.,
Yan
S.,
Hu
P.,
Huang
J.,
Zhang
G.,
Chaetocochin J, an epipolythiodioxopiperazine alkaloid, induces apoptosis and autophagy in colorectal cancer via AMPK and PI3K/AKT/mTOR pathways. Bioorganic Chemistry.
2021;
109
:
104693
.
View Article PubMed Google Scholar -
Deleyto-Seldas
N.,
Efeyan
A.,
The mTOR - autophagy axis and the control of metabolism. Frontiers in Cell and Developmental Biology.
2021;
9
:
655731
.
View Article PubMed Google Scholar -
Wang
S.,
Li
H.,
Yuan
M.,
Fan
H.,
Cai
Z.,
Role of AMPK in autophagy. Frontiers in Physiology.
2022;
13
:
1015500
.
View Article PubMed Google Scholar -
Bui
K.D.,
Pham
P. Van,
The relationship between autophagy and multidrug resistance in cancer stem cells. Science and Technology Development Journal.
2022;
25
(4)
:
2625-36
.
View Article Google Scholar -
Lefort
S.,
Joffre
C.,
Kieffer
Y.,
Givel
A.M.,
Bourachot
B.,
Zago
G.,
Inhibition of autophagy as a new means of improving chemotherapy efficiency in high-LC3B triple-negative breast cancers. Autophagy.
2014;
10
(12)
:
2122-42
.
View Article PubMed Google Scholar -
Guo
X.L.,
Li
D.,
Hu
F.,
Song
J.R.,
Zhang
S.S.,
Deng
W.J.,
Targeting autophagy potentiates chemotherapy-induced apoptosis and proliferation inhibition in hepatocarcinoma cells. Cancer Letters.
2012;
320
(2)
:
171-9
.
View Article PubMed Google Scholar -
Liu
D.,
Yang
Y.,
Liu
Q.,
Wang
J.,
Inhibition of autophagy by 3-MA potentiates cisplatin-induced apoptosis in esophageal squamous cell carcinoma cells. Medical Oncology (Northwood, London, England).
2011;
28
(1)
:
105-11
.
View Article PubMed Google Scholar -
Li
J.,
Hou
N.,
Faried
A.,
Tsutsumi
S.,
Kuwano
H.,
Inhibition of autophagy augments 5-fluorouracil chemotherapy in human colon cancer in vitro and in vivo model. European Journal of Cancer (Oxford, England).
2010;
46
(10)
:
1900-9
.
View Article PubMed Google Scholar
Comments
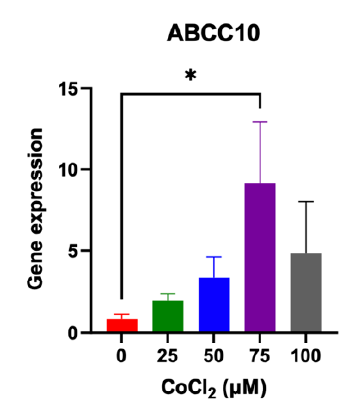
Article Details
Volume & Issue : Vol 11 No 10 (2024)
Page No.: 6859-6868
Published on: 2024-10-31
Citations
Copyrights & License

This work is licensed under a Creative Commons Attribution 4.0 International License.
Search Panel
Pubmed
Google Scholar
Pubmed
Google Scholar
Pubmed
Search for this article in:
Google Scholar
Researchgate
- HTML viewed - 205 times
- PDF downloaded - 67 times
- XML downloaded - 16 times