Abstract
Introduction: The viability of mesenchymal stem cells (MSCs) during intravenous infusion can significantly impact treatment efficacy, particularly with thawed cryopreserved MSCs. During infusion, these thawed MSCs are suspended in saline at room temperature, which lacks nutrients and buffering capacity, leading to a gradual decline in cell viability. This study aimed to investigate the survival of thawed MSCs during intravenous infusion and propose an optimal procedure to maximize cell viability.
Methods: Human MSCs derived from adipose tissues (ADSCs) and umbilical cords (UCMSCs) were utilized in this study. The MSCs were cryopreserved using a cryoprotectant-free medium (MSCCryosave OTS) at -196°C at a concentration of 107 cells/mL. They were then naturally thawed at room temperature. The 15.107 thawed MSCs in MSCCryosave OTS were suspended in 500 mL Lactated Ringer solution. The bottle was connected to an infusion tube, with the valve opened at a rate of 25 drops/min. Cell viability was assessed every 30 minutes until the bags were depleted. In another experiment, cell viability was evaluated using a phosphate-buffered saline (PBS) solution supplemented with 5% human serum albumin (the solution named CellCarrier). Cell viability was determined using a hemocytometer with trypan blue staining.
Results: The results indicated that the cell viability of thawed ADSCs and UCMSCs gradually decreased over time. For ADSCs, the percentage of viable cells decreased from 92.95 ± 1.33% post-thaw (at 0 h) to 80.41 ± 2.02% at 90 minutes of transfusion. For UCMSCs, the percentage decreased from 94.44 ± 1.69% post-thaw (at 0h) to 81.12 ± 2.26% at 90 minutes of transfusion. At the last drop of the bag, the percentage of viable ADSCs and UCMSCs was 48.22 ± 14.08% and 59.39 ± 14.54%, respectively, after approximately 385 minutes of transfusion. Notably, cell viability significantly increased when cells were infused with CellCarrier, remaining above 90% after 385 minutes (90.13 ± 0.24% for UCMSCs and 90.09 ± 0.44% for ADSCs).
Conclusion: This study suggests that using Lactated Ringer's solution results in a rapid decline in cell viability. Therefore, reducing the transfusion time is crucial to maintaining cell viability before administration to patients. We recommend keeping the transfusion time under 90 minutes when using Lactated Ringer's or alternatively utilizing CellCarrier solution for infusion.
Introduction
Mesenchymal stem cells (MSCs) are extensively utilized not only for disease treatment but also for anti-aging and longevity purposes1, 2, 3, 4, 5. This widespread application is attributed to their unique characteristics that make them suitable for treating various pathologies and combating aging processes. Specifically, MSCs can modulate the immune system, reduce inflammation, inhibit apoptosis, stimulate angiogenesis, and differentiate into multiple types of functional cells6, 7, 8, 9. Notably, MSCs lack HLA-DR expression and exhibit low immunogenicity, which makes them ideal for allogeneic use9, 10, 11. In recent years, "off-the-shelf" allogeneic MSC products have been developed in cryopreserved forms, readily available for use12, 13, 14.
Despite facilitating the clinical application of off-the-shelf cyropreserved MSCs, some studies have reported that thawed MSCs may have reduced potency compared to fresh cells. François et al. (2012) indicated that cryopreserved MSCs might experience impaired immunosuppressive properties due to heat-shock response and diminished interferon-γ licensing15. Additionally, Moll et al. (2014) observed that thawed MSCs exhibited reduced immunomodulatory and blood regulation properties16. In another study, IFNγ-prelicensed thawed MSCs demonstrated less lung tropism in vivo following intravenous injection compared to fresh MSCs17. In contrast, Cruz et al. (2015) reported that thawed bone marrow MSCs were as effective as fresh cells in a murine model of allergic airway inflammation18. Similarly, Tan et al. (2019) found that thawed MSCs retained comparable potency to fresh cells both in vitro and in a polymicrobial septic animal model19.
While most studies focus on evaluating the survival rate of MSCs post-thawing, no research has yet assessed the survival of thawed MSCs over time during intravenous infusion. This study aims to ascertain the survival of thawed MSCs when suspended in infusion solutions.
Methods
Expansion of ADSCs and UCMSCs
Adipose-derived stem cells (ADSCs) and umbilical cord-derived mesenchymal stem cells (UCMSCs) were sourced from the Cellbank at the Stem Cell Institute, University of Science, Vietnam National University, Ho Chi Minh City (VNUHCM), Vietnam. ADSCs were thawed and cultured in ADSCCult I medium (Regenmedlab, Ho Chi Minh City, Vietnam), while UCMSCs were thawed and cultured in MSCult I medium (Regenmedlab, Ho Chi Minh City, Vietnam). Subculturing of these cells was performed using the enzyme Deattachment (Regenmedlab, Ho Chi Minh City, Vietnam) at a splitting ratio of 1:3.
Crypropreservation and thawing
ADSCs and UCMSCs were harvested and cryopreserved in MSCCryosave OTS medium, a DMSO-free cryopreservation medium (Regenmedlab, Ho Chi Minh City, Vietnam), at a concentration of 107 cells/mL per cryovial. The cryovials were cooled at a rate of 1°C per minute in a freezing box and stored overnight at -86°C. All vials were subsequently transferred to liquid nitrogen tanks the following day.
The thawing process was conducted according to the manufacturer’s guidelines. Briefly, in step 1, the cryovials containing ADSCs and UCMSCs were transferred from the liquid nitrogen tank to a -86°C freezer overnight. In step 2, the vials were placed at room temperature to allow for natural thawing. The ice in the vials completely thawed within 5 to 10 minutes. Once the ice was completely dissolved, the cell suspension was mixed with Afterfreeze solution (Regenmedlab, HCMC, VN) in a 1:1 ratio (cell suspension: Afterfreeze solution). The thawed vials were then used in subsequent experiments.
Experimental design to determine the cell viability of ADSCs and UCMSCs during infusion
A 30 mL thawed cell suspension, comprising 15 mL of MSCCryosave OTS and 15 mL of Afterfreeze from 15 vials, was administered into a 500 mL Lactated Ringer's solution bottle. The infusion tube was attached to this bottle, and the flow rate was adjusted to 25 drops per minute. Approximately 20-30 drops were collected every 30 minutes to assess cell viability (Figure 1). The experiments were conducted in triplicate.
Cell viability
Cell viability was assessed using a hemocytometer with trypan blue staining. To evaluate cell viability using Trypan Blue and a hemocytometer, harvested cells were first suspended in a liquid medium. The cell suspension was then mixed with an equal volume of 0.4% Trypan Blue solution. The hemocytometer was cleaned, and a coverslip was placed over it. The stained cell mixture was loaded into the chamber, allowing it to distribute by capillary action. Under low magnification on a microscope, cells were counted on the grid: clear cells were counted as viable, while blue-stained cells were considered non-viable. For accuracy, a minimum of 100 cells was counted. Viability was calculated by dividing the number of living cells by the total number of cells and adjusting for the dilution factor.
Statistical analysis
Each experiment was independently repeated three times. Results are expressed as the mean ± standard deviation (SD). Statistical significance was determined at p < 0.05. Data processing and plotting were performed using Prism software.
Results
The viability of MSCs gradually decrease following time during the dropping
The data depicted in Figure 2 demonstrate that ADSCs suspended in Ringer lactate solution exhibit a progressive decline in viability as the infusion progresses. Specifically, after 90 minutes of infusion, the viability rate of the cells dropped to 80.41 ± 2.02%, from an initial rate of 92.95 ± 1.33% at 0 minutes (p < 0.05). Following the completion of a 530 mL infusion at 385 minutes, the viability rate further diminished to 48.22 ± 14.08%. Linear regression analysis reveals that the viability rate declines over time according to the equation y = -0.1168x + 91.506 (R² = 0.9945).
Similarly, UCMSC cells exhibit a declining survival rate over time (Figure 3). Initially, at 0 minutes, the cell survival rate was 94.44 ± 1.69%. This rate decreased to 81.12 ± 2.26% following 90 minutes of infusion and further declined to 59.39 ± 14.54% at 385 minutes (p < 0.05). After the infusion of 530 mL over 385 minutes, the survival rate remained quite low at 59.39 ± 14.54%. Linear regression analysis indicated that the cell survival rate decreased over time, following the equation y = -0.0902x + 90.585 (R² = 0.958).
The viability of MSCs is significantly improved in CellCarrier
The viability rate of MSCs, including ADSCs and UCMSCs, significantly increases when they are infused in a CellCarrier solution. For UCMSCs, there is no significant change in cell viability during the initial 30 minutes (89.77 ± 3.88 in Lactated Ringer vs. 93.87 ± 1.90 in CellCarrier, p > 0.05). However, at 60 minutes, the viability rates significantly differ between cells suspended in Lactated Ringer solution and those in CellCarrier solution (85.03 ± 3.22 in Lactated Ringer vs. 92.41 ± 1.78 in CellCarrier, p < 0.05). This difference becomes more pronounced from 90 minutes to 385 minutes (Figure 4A). At 385 minutes, the viability rate of UCMSCs in CellCarrier solution reaches 90.09 ± 0.44%, in contrast to only 49.32 ± 15.31% in Lactated Ringer solution (p < 0.01).
ADSCs exhibit a survival pattern similar to UCMSCs. As illustrated in Figure 4B, the survival rates of ADSCs are comparable in both Lactated Ringer and CellCarrier solutions during the initial 30 minutes (90.77 ± 4.08% in Lactated Ringer vs. 92.87 ± 1.80% in CellCarrier; p > 0.05). However, from 60 to 385 minutes, the survival rate in the CellCarrier solution is significantly higher than in the Lactated Ringer solution (p < 0.05). Specifically, at minute 60, the survival rate in the Lactated Ringer solution is 88.03 ± 4.12%, whereas it is 92.41 ± 1.58% in the CellCarrier solution (p < 0.05). By minute 385, the survival rate drops to 50.32 ± 13.31% in the Lactated Ringer solution, while it remains at 90.13 ± 0.24% in the CellCarrier solution (p < 0.01).
Discussion
Mesenchymal stem cell therapy is currently among the most widely used forms of stem cell therapies. Mesenchymal stem cells derived from adipose tissue (ADSCs) and those sourced from umbilical cord tissue (UCMSCs) are employed in treating various conditions, including chronic obstructive pulmonary disease, diabetes, autoimmune diseases, and stroke1, 2, 3, 4, 5. Unlike certain other stem cell types, MSCs demonstrate low HLA-DR expression and minimal immunogenicity, which facilitates their use in allogeneic transplantation9, 10, 11. Consequently, off-the-shelf cryopreserved MSC products have been developed for immediate application. Although the post-thaw viability of these cells has been extensively studied, their survival during infusion over time has not been thoroughly explored. This study is the first to examine the survival of thawed MSCs throughout the infusion period, with a focus on UCMSCs and ADSCs. Preliminary research suggests that both the duration of infusion and the choice of infusion solution considerably affect the survival of thawed mesenchymal stem cells.
The study's findings indicate that MSCs, when thawed and stored in Ringer lactate solution, experience rapid cell death during infusion at room temperature. Specifically, both UCMSCs and ADSCs exhibited over 20% cell death after 90 minutes of infusion. This suggests that Ringer lactate solution is inadequate for sustaining MSC viability over extended periods. Contrasting these findings, Ngo et al. (2021) reported that Ringer lactate could maintain high survival rates for ADSC and UCMSC, with more than 70% viability at room temperature after 72 hours20. The key difference between our study and that of Ngo et al. lies in the cell condition; our study utilized frozen and thawed cells, whereas Ngo et al. (2021) employed fresh cells. Sultana et al. (2022) recommended storing ADSCs in carrier solutions like saline, 5% DS, Hepa-Sal, or HS at 4 °C, ideally for less than 6 hours and no more than 12 hours21. Furthermore, Kubrova et al. (2020) demonstrated a significant reduction in ADSC cell number and metabolic activity after 4 hours at three temperatures studied (4 °C, 23 °C, and 37 °C)22.
The process of freezing and thawing inevitably inflicts damage on MSCs. Post-thawing, when MSCs are suspended in Lactated Ringer solution, they may continue to experience membrane damage, resulting in cell death. In our subsequent research efforts, we enhanced the thawing medium by employing CellCarrier solution, which is a phosphate-buffered saline (PBS) supplemented with 5% human albumin. Our findings indicate that PBS with 5% human albumin significantly improves the survival rate of cells post-90 minutes of thawing. Although the survival rate of MSCs, UCMSCs and ADSCs, gradually declines after 90 minutes of infusion, the rate remains above 90% until the infusion concludes at 385 minutes. This observation mirrors findings related to hematopoietic stem cells derived from cord blood23. Lachica et al. (2023) identified PBS augmented with 5% human albumin as a suitable medium for the preservation of thawed hematopoietic stem cells, wherein mononuclear cells (MNCs) remain stable for up to 4 hours, and CD34+ hematopoietic stem cells maintain stability for up to 6 hours. They confirmed that cord blood hematopoietic stem cells can be satisfactorily washed and stabilized in PBS with 5% human albumin up to 4 hours post-thawing23.
Hence, this study underscores the necessity of considering post-thaw utilization of off-the-shelf MSC products intended for therapeutic applications. The choice of storage solution post-thawing significantly influences the viability of MSCs. To mitigate cell death during infusion, it is imperative to reduce the infusion duration and employ an appropriate infusion medium, such as PBS with 5% human albumin. Furthermore, for optimal therapeutic efficacy, the dosage should be calculated based on the actual number of viable cells during infusion.
Conclusion
The study evaluates the viability of thawed MSCs derived from umbilical cords and adipose tissue during infusion with Lactated Ringer solution. Although Lactated Ringer is traditionally used for MSC infusions, it may be suboptimal for thawed cells due to an increased cell mortality rate over time. The findings suggest that using phosphate-buffered saline (PBS) supplemented with 5% human albumin significantly improves cell viability during infusion. These preliminary results recommend that thawed MSCs should meticulously choose appropriate infusion solutions to ensure the delivery of a viable cell dosage.
Abbreviations
ADSCs - Adipose-derived Stem Cells, DMSO - Dimethyl Sulfoxide, HLA-DR - Human Leukocyte Antigen-DR isotype, MNCs - Mononuclear Cells, MSCs - Mesenchymal Stem Cells, PBS - Phosphate-Buffered Saline, UCMSCs - Umbilical Cord-derived Mesenchymal Stem Cells
Acknowledgments
We thank the Cellbank of Stem Cell Institute, University of Science, VNUHCM, Ho Chi Minh City, Viet Nam, for providing ADSCs and UCMSCs for this study.
Author’s contributions
Pham Van Phuc (PVP): Conceived and designed the experiments; analyzed cell phenotypes; analyzed the data; wrote the manuscript. Phan Tuan Kiet (PTK), and Nguyen Thi Hien Trang (NTHT): Performed the experiments; analyzed and interpreted the data. Vu Bich Ngoc (VBN), Le Thi Bich Phuong (LTBP): Assisted with the design of the study and provided critical feedback on the manuscript; participated in the research design and helped to draft the manuscript; performed the experiment. All authors read and approved the final manuscript.
Funding
None.
Availability of data and materials
Data and materials used and/or analyzed during the current study are available from the corresponding author on reasonable request.
Ethics approval and consent to participate
Not applicable.
Consent for publication
Not applicable.
Competing interests
The authors declare that they have no competing interests.
References
-
Abouzid
M.R.,
Ali
K.,
Kamel
I.,
Esteghamati
S.,
Saleh
A.,
Ghanim
M.,
The Safety and Efficacy of Human Umbilical Cord-Derived Mesenchymal Stem Cells in Patients With Heart Failure and Myocardial Infarction: A Meta-Analysis of Clinical Trials. Cureus.
2023;
15
(11)
:
e49645
.
View Article PubMed Google Scholar -
Swain
H.N.,
Boyce
P.D.,
Bromet
B.A.,
Barozinksy
K.,
Hance
L.,
Shields
D.,
Mesenchymal stem cells in autoimmune disease: A systematic review and meta-analysis of pre-clinical studies. Biochimie.
2024;
223
:
54-73
.
View Article PubMed Google Scholar -
Irani
D.,
Mehrabani
D.,
Karimi-Busheri
F.,
Mesenchymal Stem Cells in Regenerative Medicine, Possible Applications in The Restoration of Spermatogenesis: A Review. Cell Journal.
2024;
26
(3)
:
169-84
.
PubMed Google Scholar -
Zhidu
S.,
Ying
T.,
Rui
J.,
Chao
Z.,
Translational potential of mesenchymal stem cells in regenerative therapies for human diseases: challenges and opportunities. Stem Cell Research & Therapy.
2024;
15
(1)
:
266
.
View Article PubMed Google Scholar -
Zhu
Y.,
Huang
C.,
Zheng
L.,
Li
Q.,
Ge
J.,
Geng
S.,
Safety and efficacy of umbilical cord tissue-derived mesenchymal stem cells in the treatment of patients with aging frailty: a phase I/II randomized, double-blind, placebo-controlled study. Stem Cell Research & Therapy.
2024;
15
(1)
:
122
.
View Article PubMed Google Scholar -
Ebrahimi
F.,
Pirouzmand
F.,
Pecho
R.D. Cosme,
Alwan
M.,
Mohamed
M. Yassen,
Ali
M.S.,
Application of mesenchymal stem cells in regenerative medicine: A new approach in modern medical science. Biotechnology Progress.
2023;
39
(6)
:
e3374
.
View Article PubMed Google Scholar -
Li
J.,
Huang
Y.,
Sun
H.,
Yang
L.,
Mechanism of mesenchymal stem cells and exosomes in the treatment of age-related diseases. Frontiers in Immunology.
2023;
14
:
1181308
.
View Article PubMed Google Scholar -
Al-Ghadban
S.,
Bunnell
B.A.,
Adipose Tissue-Derived Stem Cells: Immunomodulatory Effects and Therapeutic Potential. Physiology (Bethesda, MD).
2020;
35
(2)
:
125-33
.
View Article PubMed Google Scholar -
Schu
S.,
Nosov
M.,
O'Flynn
L.,
Shaw
G.,
Treacy
O.,
Barry
F.,
Immunogenicity of allogeneic mesenchymal stem cells. Journal of Cellular and Molecular Medicine.
2012;
16
(9)
:
2094-103
.
View Article PubMed Google Scholar -
Kuçi
Z.,
Piede
N.,
Vogelsang
K.,
Pfeffermann
L.M.,
Wehner
S.,
Salzmann-Manrique
E.,
Expression of HLA-DR by mesenchymal stromal cells in the platelet lysate era: an obsolete release criterion for MSCs?. Journal of Translational Medicine.
2024;
22
(1)
:
39
.
View Article PubMed Google Scholar -
Consentius
C.,
Reinke
P.,
Volk
H.D.,
Immunogenicity of allogeneic mesenchymal stromal cells: what has been seen in vitro and in vivo?. Regenerative Medicine.
2015;
10
(3)
:
305-15
.
View Article PubMed Google Scholar -
Lynggaard
C.D.,
Gr∅nh∅j
C.,
Christensen
R.,
Fischer-Nielsen
A.,
Melchiors
J.,
Specht
L.,
Intraglandular Off-the-Shelf Allogeneic Mesenchymal Stem Cell Treatment in Patients with Radiation-Induced Xerostomia: A Safety Study (MESRIX-II). Stem Cells Translational Medicine.
2022;
11
(5)
:
478-89
.
View Article PubMed Google Scholar -
Kubota
H.,
Arakawa
Y.,
Mizushima
Y.,
Irikura
T.,
Watakabe
M.,
Ishikawa
T.,
Efficacy of off-the-shelf bone marrow mesenchymal stem cells for pediatric steroid-refractory acute graft-versus-host disease. Blood Cell Therapy.
2023;
7
(1)
:
1-9
.
PubMed Google Scholar -
Montemurro
T.,
Lavazza
C.,
Montelatici
E.,
Budelli
S.,
La Rosa
S.,
Barilani
M.,
Off-the-Shelf Cord-Blood Mesenchymal Stromal Cells: Production, Quality Control, and Clinical Use. Cells.
2024;
13
(12)
:
1066
.
View Article PubMed Google Scholar -
François
M.,
Copland
I.B.,
Yuan
S.,
Romieu-Mourez
R.,
Waller
E.K.,
Galipeau
J.,
Cryopreserved mesenchymal stromal cells display impaired immunosuppressive properties as a result of heat-shock response and impaired interferon-γ licensing. Cytotherapy.
2012;
14
(2)
:
147-52
.
View Article PubMed Google Scholar -
Moll
G.,
Alm
J.J.,
Davies
L.C.,
von Bahr
L.,
Heldring
N.,
Stenbeck-Funke
L.,
Do cryopreserved mesenchymal stromal cells display impaired immunomodulatory and therapeutic properties?. Stem Cells (Dayton, Ohio).
2014;
32
(9)
:
2430-42
.
View Article PubMed Google Scholar -
Chinnadurai
R.,
Copland
I.B.,
Garcia
M.A.,
Petersen
C.T.,
Lewis
C.N.,
Waller
E.K.,
Cryopreserved Mesenchymal Stromal Cells Are Susceptible to T-Cell Mediated Apoptosis Which Is Partly Rescued by IFNγ Licensing. Stem Cells (Dayton, Ohio).
2016;
34
(9)
:
2429-42
.
View Article PubMed Google Scholar -
Cruz
F.F.,
Borg
Z.D.,
Goodwin
M.,
Sokocevic
D.,
Wagner
D.,
McKenna
D.H.,
Freshly thawed and continuously cultured human bone marrow-derived mesenchymal stromal cells comparably ameliorate allergic airways inflammation in immunocompetent mice. Stem Cells Translational Medicine.
2015;
4
(6)
:
615-24
.
View Article PubMed Google Scholar -
Tan
Y.,
Salkhordeh
M.,
Wang
J.P.,
McRae
A.,
Souza-Moreira
L.,
McIntyre
L.,
Thawed Mesenchymal Stem Cell Product Shows Comparable Immunomodulatory Potency to Cultured Cells In Vitro and in Polymicrobial Septic Animals. Scientific Reports.
2019;
9
(1)
:
18078
.
View Article PubMed Google Scholar -
Ngo
A.T.,
Le
H.M.,
Trinh
N.T.,
Jun
A.P.,
Bach
T.Q.,
Bui
H.T.,
Clinically relevant preservation conditions for mesenchymal stem/stromal cells derived from perinatal and adult tissue sources. Journal of Cellular and Molecular Medicine.
2021;
25
(22)
:
10747-60
.
View Article PubMed Google Scholar -
Sultana
T.,
Dayem
A.A.,
Lee
S.B.,
Cho
S.G.,
Lee
J.I.,
Effects of carrier solutions on the viability and efficacy of canine adipose-derived mesenchymal stem cells. BMC Veterinary Research.
2022;
18
(1)
:
26
.
View Article PubMed Google Scholar -
Kubrova
E.,
Qu
W.,
Galvan
M.L.,
Paradise
C.R.,
Yang
J.,
Dietz
A.B.,
Hypothermia and nutrient deprivation alter viability of human adipose-derived mesenchymal stem cells. Gene.
2020;
722
:
144058
.
View Article PubMed Google Scholar -
Lachica
C.A.,
Miele
M.J.,
Herrera
S.M.,
Elanbari
M.,
Deola
S.,
Saleh
A.,
Albumin-based solution is the ideal post-thawing suspension medium for cord blood hematopoietic stem cells: A stability and proliferative evaluation. Transfusion.
2023;
63
(5)
:
1050-9
.
View Article PubMed Google Scholar
Comments
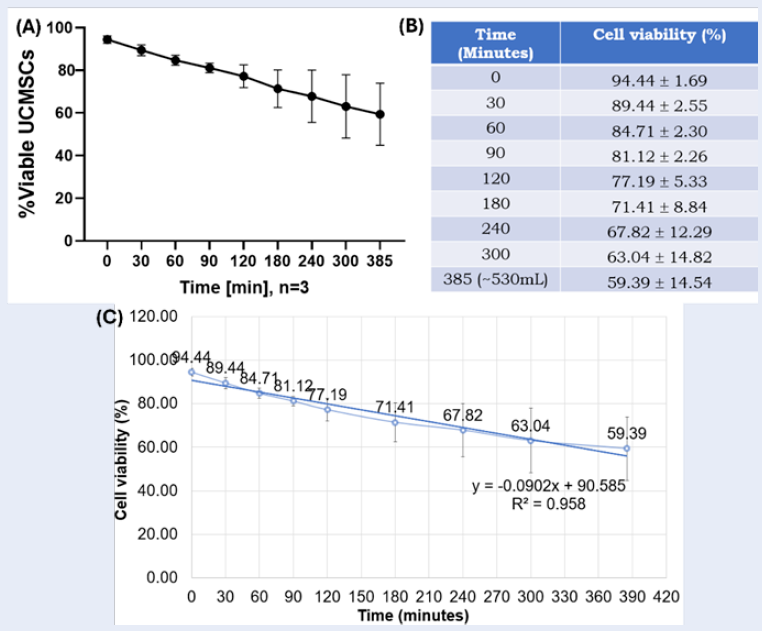
Article Details
Volume & Issue : Vol 11 No 9 (2024)
Page No.: 6730-6736
Published on: 2024-09-30
Citations
Copyrights & License

This work is licensed under a Creative Commons Attribution 4.0 International License.
Search Panel
Pubmed
Google Scholar
Pubmed
Google Scholar
Pubmed
Google Scholar
Pubmed
Google Scholar
Pubmed
Search for this article in:
Google Scholar
Researchgate
- HTML viewed - 1628 times
- PDF downloaded - 576 times
- XML downloaded - 70 times