Production of dendritic cells and cytokine-induced killer cells from banked umbilical cord blood samples
Abstract
Umbilical cord blood (UCB) is considered to be a source of hematopoietic stem cells (HSCs). All UCB banks have recently become interested in the isolation and storage of HSCs for the treatment of hematological diseases. However, UCB was also recently confirmed as a source of immune cells for immunotherapy such as dendritic cells (DCs) and cytokine-induced killer cells (CIKs). This study aimed to exploit this source of immune cells in banked UCB samples. After collection of UCB samples, mononuclear cells (MNCs) containing stem cells, progenitor cells, and mature cells were isolated by Ficoll-Hypaque-based centrifugation. The MNCs were subjected to freezing and thawing according to a previously published protocol. The banked MNCs were used to produce DCs and CIKs. To produce DCs, MNCs were induced in RPMI 1640 medium supplemented with GM-CSF (50 ng/ml) and IL-4 (40 ng/ml) for 14 days. To produce CIKs, MNCs were induced in RPMI 1640 medium supplemented an anti-CD3 monoclonal antibody, IL-3, and GMC-SF for 21–28 days. Both DCs and CIKs were evaluated for their phenotypes and functions according to previously published protocols. The results showed that banked UCB samples can be successfully used to produce functional DCs and CIKs. These samples are valuable sources of immune cells for immunotherapy. The present results suggest that banked UCB samples are useful not only for stem cell isolation, but also for immune cell production.
Introduction
Umbilical cord blood (UCB) is considered to be a source of stem cells, especially hematopoietic stem cells (HSCs). Transplantation of UCB-derived HSCs in the treatment of hematological diseases has been performed for a long time. The first cord blood (CB) transplantation was performed in 1988 in a patient with Fanconi anemia Gluckman et al., 1989. Banked UCB samples have also been used to treat refractory leukemia at Duke University Medical Center since 1993 Kurtzberg et al., 1996.
In recent years, many UCB banks have been established around the world. The Netcord group was created in 1998 to establish good practices in UCB storage. The inventory of the Netcord group, a cooperative network of large experienced UCB banks, currently contains more than 200 000 cryopreserved UCB units ready for clinicaluse Gluckman, 2009. However, the usage efficiency of these banks is very low. In fact, some private banks only use UCB samples for the donors, while some public banks are faced with seeking HLA-matched samples. For these reasons, some studies and banks have exploited other kinds of stem cells in banked UCB samples. Recently, mesenchymal stem cells (MSCs) were successfully isolated from banked UCBs Phuc et al., 2012Phuc et al., 2011.
Fresh UCB samples have been used to produce certain kinds of immune cells for treatment, especially dendritic cells (DCs) Kim et al., 2015Liu et al., 2015Park et al., 2015 and cytokine-induced killer cells (CIKs) Durrieu et al., 2013Niu et al., 2011Zhang et al., 2014. The authors isolated DCs from fresh UCB samples. These DCs clearly exhibited the particular DC phenotypes. The above studies showed that fresh UCB samples comprise a useful source of DCs for clinical applications. Moreover, some recent publications have shown that mononuclear cells (MNCs) from UCB samples can differentiate into functional CIKs Durrieu et al., 2013Niu et al., 2011Zhang et al., 2014. These CIKs acted as natural killer (NK) cells both in vitroand in vivo Dalle et al., 2005Fan et al., 2008Verneris and Miller, 2009. Furthermore, UCBderived NK cells express less granzyme and are thus less cytotoxic than peripheral blood-derived NK cells Wang et al.,2007. These cells were successfully used in certain treatments, such as relapse of acute myeloid leukemia after HLA-mismatched haploidentical stem cell transplantation Hanley et al., 2010Ruggeri et al., 2007, esophageal cancer Wang et al., 2014, hepatocellular carcinoma, renal cell carcinoma, and lung cancer (NCT01914263).
To increase the usage efficiency of banked UCB samples, this study aimed to produce DCs and CIKs from banked UCB samples for immunotherapy. Both DCs and CIKs were produced from UCB-derived MNCs and tested for their phenotypes and in vitro activities.
Materials and methods
UCB collection and MNC isolation
Umbilical cord blood was collected from a healthy pregnant woman with agreement among the hospital, donor, and laboratory. After the woman gave birth, blood was taken from the fetal cord and treated with the anticoagulant CDPA (Terumo, Japan).
To isolate MNCs, each UCB unit was diluted with phosphate-buffered saline (PBS) at a ratio of 1:1, and 10 ml of the diluted blood was gently loaded onto 25 ml of Ficoll-Hypaque solution (1.077 g/ml; Sigma– Aldrich, St Louis, MO) in a 50-ml tube. After centrifugation at 2,500 rpm for 5 min, MNCs were derived from the interphase layer (above the Ficoll-Hypaque layer), and washed twice with PBS.
MNC banking and thawing
The isolated MNCs were resuspended in IMDM cryomedium (Sigma–Aldrich) supplemented with 10% DMSO (Sigma–Aldrich) and 20% fetal bovine serum (FBS) at the density of 107–108 cells/ml. The sample was transferred to a controlled-rate freezer precooled to 0°C, and sequentially cooled at 1°C/min to −12°C, cooled at 20°C/min to −60°C, warmed at 15°C/min to −18°C, cooled at 1°C/min to −60°C, and cooled at 3°C/min to −100°C. After completion of the freezing protocol, the units were stored in liquid nitrogen.
The sample was rapidly thawed by embedding the cryotube in a 37°C water bath until it was completely liquefied. Immediately afterward, 1 ml of IMDM with 20% FBS and 1% antibiotic/mycotic solution was dropped into the tube. The cell suspension was then transferred to a new 15-ml tube, and centrifuged at 2,500 rpm for 5 min to eliminate the DMSO. The cell pellet was resuspended in culture medium (IMDM plus 20% FBS and 1% antibiotic/mycotic solution) for use in further experiments.
DC production and characterization
MNCs were cultured in IMDM medium supplemented with 10% FBS at 37°C for 2–3 h. The adherent cells were cultured to allow their differentiation into DCs according to the following procedures. MNCs were cultured for 9 days in RPMI 1640 medium (Roswell Park Memorial Institute) containing 40 ng/ml interleukin (IL)-4, 50 ng/ml GM-CSF (Santa Cruz Biotechnology, Santa Cruz, CA), and 10% FBS. Fresh medium with added cytokines was additionally provided after a 3-day interval to provide nutrients.
Before and after induction, surface markers of DCs were analyzed by flow cytometry to determine whether DCs were successfully differentiated. Specific primary antibodies were used, such as those against CD14, CD1a, CD80, and CD83 (Santa Cruz Biotechnology). DCs were incubated in 100 μl of PBS containing 5 μl of each monoclonal antibody for 20 min in the dark at room temperature. The cells were then washed three times in PBS and analyzed using FACS flow cytometry (FACSCalibur; Becton Dickinson, Heidelberg, Germany) and Cell Quest software.
CIK production and characterization
Generation of CIKs
MNCs were cultured in serum-free X-VIVO-15 medium (BioWhittaker, Walkersville, MD) with 1000 U/ml of IFN-γ (Gammakine; Boehringer Ingelheim, Vienna, Austria) added on day 0, 50 ng/ml of anti-CD3 antibody (OKT-3; Janssen-Cilag SpA, Milan, Italy) added on day 1, and 500 U/ml of rhIL-2 included in the medium from day 1 onward. Cell expansion was performed for 21–28 days.
CIK phenotype analysis
CIKs were characterized using FITC-conjugated anti- CD3 (SK7 clone) and PE-conjugated anti-CD56 (NCAM16.2 clone) monoclonal antibodies (mAbs) (Becton Dickinson). To analyze the expression of activating receptors on CIKs, the following mAbs were used: anti-NKG2D (BAT221 clone) and anti-NKp30 (A76 clone).
For indirect immunofluorescence staining, cells were incubated with the primary mAbs, followed by PEconjugated goat anti-mouse IgG1 (Invitrogen, Paisley, UK) or FITC-conjugated goat anti-mouse Ig (Becton Dickinson) secondary antibodies. A FACSCalibur flow cytometer (Becton Dickinson) was used to analyze the samples.
Cell conjugation assay
The target cells (MCF7) were washed, resuspended in 1 ml of PBS supplemented with 1% bovine serum albumin at a final concentration of 5 × 106 cells/ml, and labeled with the green fluorescent dye carboxyfluorescein succinimidyl ester (CFSE; 2 μM; Sigma– Aldrich) for 10 min at 37°C. Quench-staining was performed on ice for 5 min by adding 5 volumes of icecold complete RPMI. The cells were then washed three times with ice-cold PBS containing 1% bovine serum albumin and cultured under appropriate conditions.
For PKH26 staining of CIKs, 10 × 106 cells were washed in PBS and the cell pellet was resuspended in 500 μL of diluent C (Sigma–Aldrich). The lipophilic red fluorescent dye PKH26 (Sigma-Aldrich) was diluted at a final concentration of 4 μM in 500 μl of diluent C, rapidly added to the cells, and incubated for 5 min at room temperature with occasional agitation. Next, 1 ml of FBS was added and incubated for 1 min at room temperature. The cells were then washed three times in complete culture medium.
A total of 5 × 105 PKH26-labeled CIKs and 5 × 105 CFSE-labeled target cells were resuspended in 200 μl of complete medium containing or lacking 1 mM ethylenediaminetetraacetic acid (EDTA; Sigma–Aldrich) and mixed in a 12-mm × 75-mm polystyrene tube (Becton Dickinson Labware, Franklin Lakes, NJ). The effector- target cell mixture was centrifuged at 1,000 rpm for 1 min and incubated at 37°C for specified time periods (5 min and 15 min). The cells were then gently resuspended and analyzed by flow cytometry.
Calcein release cytotoxicity assay
The target cells (MCF7, PC3, and HepG2) were labeled with 3.5 μM calcein-acetoxymethyl ester (calcein-AM; Sigma–Aldrich) for 30 min at 37°C. The labeled cells were then added to 96-well plates at 5 × 103 cells/well. CIKs were added at different effector-to-target (E:T) ratios. In control samples, the cytotoxicity assay was performed in the presence of 1 mM EDTA. After 4 h, the cells were sedimented by centrifugation. Next, 100 μl of supernatant was collected and determined for calcein release using a fluorescence microplate reader with excitation at 485 nm and emission at 535 nm. The percentage of specific calcein release was calculated using the following formula: percent specific lysis = (test release minus spontaneous release) × 100 / (maximal release minus spontaneous release). Maximal lysis was achieved with 1% Triton X-100.
Results
High percentage of viable cells after thawing
Ten banked UCB samples were thawed and used for induction of DCs and CIKs. Before use, all thawed samples were tested for the percentage of viable cells. The results showed that there were 91.5 ± 4.56% viable cells in all thawed samples. Each sample was divided into two parts, half for DC induction and half for CIK induction.
Banked UCBs successfully produce functional DCs
Induced cells exhibit the DC phenotype
Thawed UCB-derived MNCs successfully exhibited the DC phenotype. In the adherent culture after 14 days of induction, the cells expressed a similar shape to DCs with many dendrites ( Figure 1 ). Regarding surface marker expression, the cells expressed DC markers, including CD83 (35.27 ± 18.7%), CD1a (18.9 ± 7.1%), and CD80 (25.88 ± 11.01%), while CD14 was not expressed in CD1a+ cells.
DC candidates can phagocytose FITC-dextran
Phagocytosis is a property of DCs. In this assay, DCs were induced in FITC-dextran-supplemented culture medium. The results revealed that the induced DCs efficiently phagocytosed the FITC-dextran. Compared with control cells incubated at 4°C, up to 42.6 ±9.5% of DCs incubated at 37°C could phagocytose FITCdextran. Meanwhile, at this temperature, about 8.7±24.5% of cells could phagocytose this dextran ( Figure 2 ).
DC candidates stimulate allogenic T cells
The induced DCs were mixed with allogenic T cells to evaluate the stimulatory potential of the DCs. The results are shown in Figure 3 . The DCs effectively stimulated the T-cell proliferation in a manner dependent on the ratio of DCs to T cells. The ratio of 1 DC to 10 T cells exhibited the highest stimulating efficacy, and the capacity gradually decreased as the ratio of DCs to T cells decreased. All results were significant compared with the control cells.
Banked UCBs successfully produce functional CIKs Induced cells exhibit CIK phenotypes
The CIK population with the CD3+CD56+ phenotype was extremely low in the banked UCB samples, and almost of the cells were CD8+. However, after 2 weeks of culture, the percentage of CD3+CD56+ cells had significantly increased to 30.56±7.89%. Besides the CD3+CD56+ population, the cultured cells were almost completely CD3+CD56- cells (45.32±12.82%). The total CD3+ cells accounted for 86.76±45% of the cultured cell population.
CIKs can bind to target cells
Binding of CIKs to target cells is an essential property of CIKs. The assay showed that CIKs were able to bind to target tumor cells. The CIKs were stained with PKH26 and the breast, lung, and liver cancer cells were stained with CFSE. In comparison with control cells (without EDTA), the CIKs bound to the three kinds of tested cancer cells. The percentages of conjugated CIK/cancer cells with double staining (PKH26 and CFSE) were maximal at 15 min after mixing(7.21% at 5 min, 28.92% at 15 min) ( Figure 4 ).
CIKs can lyse target cells
Lysis of the target cells by CIKs was recorded by calce- in assays. The results are shown in Figure 5 . At the E:T ratio of 10:1, the lysis of target cells was highest in all kinds of cancer cells (42%, 38%, and 21% for prostate cancer cells, liver cancer cells, and breast cancer cells, respectively). The percentages of lysed target cells gradually decreased as the E:T ratio decreased. Specif-ically, for PC3 prostate cancer cells, the percentage of lysis was highest at the E:T ratio of 10:1 (42%), but on¬ly 33% at the E:T ratio of 5:1 and 17% at the E:T ratio of 2.5:1. For HepG2 liver cancer cells, the percentage of lysis was highest at the E:T ratio of 10:1 (38%), and gradually decreased to 29% and 15% for E:T ratios of 5:1 and 2.5:1, respectively. Similarly, in MCF7 breast cancer cells, the percentage of lysis was highest at the E:T ratio of 10:1 (21%), and gradually decreased to 17% and 9% at E:T ratios of 5:1, and 2.5:1, respectively.
Discussion
Immunotherapy is considered to be a promising therapy for certain diseases, especially cancer. In recent years, immune cell-based immunotherapies have rapidly developed in cancer treatment. Some of the immune cells used include DCs and CIKs. The most difficult aspect of immune cell-based treatments is related to their limited sources. The results of this study suggest the possible use of banked UCB samples as sources of DCs and CIKs for immunotherapies.
In the first experiment, we standardized the UCB samples based on their HSC counts. In this manner, 100% of the banked samples were successfully cryo- preserved and thawed with a high percentage of via¬ble cells (about 90%). The thawed MNCs could be suc-cessfully induced to produce both functional DCs and CIKs.
MNCs comprise complex cocktails of certain kinds of cells like as HSCs, MSCs, EPCs, and monocytes. How-ever, the highest ratio is MNCs, with more than 90%. MNCs are easily induced to differentiate into DCs, as described in previous reports Kim et al., 2015Liu et al., 2015Park et al., 2015. In this study, we also recorded that these cells could be differentiated into functional DCs. After 7 days of induction with cytokines, and 7 days of induction with TNF-a, these cells successfully exhibited the DC phenotype, such as expression of CD1a, CD80, and CD83 and lack of expression of CD14. CD80 and CD83 are important co-stimulators of antigen presentation with T cells. More importantly, the DCs successfully produced IL-12. IL-12 is an essential interleukin for stimulation of T lymphocyte proliferation. In fact, the cell assays showed that the obtained DCs could stimulate T cell proliferation in vitro. In vivo, the role of DCs is important. In particular, after receiving antigens, they present these antigens to T cells as well as B cells. By this process, DCs can trigger T cell proliferation to attack the targets. Phagocytosis is an important characteristic of DCs. The present results showed that the DCs could phagocytose FITC-dextran. In comparisons with DCs derived from fresh UCB samples published previously, the banked UCB-derived DCs clearly exhibited similar phenotypes. In fact, in the study by Bracho et al., 2003, they successfully produced functional DCs from fresh UCB samples with 31.27 ± 11.7% CD83, 23.4 ± 4.2% CD1a, and 21.97 ± 12.01% CD80. They also recorded that the morphological DCs expressed CDla, but not CD14. In another study, Enomoto et al., 2000 compared DCs from fresh peripheral blood and cryopreserved PB, and found similar phenotypes between the two DC samples for endocytic capacity, chemotactic migratory capacities, and primary allogeneic T-cell responses.
In the next experiment, we successfully produced CIKs from banked MNC samples. In fact, the induced CIKs exhibited all the particular characteristics of CIKs, including exhibition of the CD3+CD56+ phenotype and binding to some target cells such as MCF7 breast cancer cells, PC3 prostate cancer cells, and HepG2 liver carcinoma cells. In particular, these CIKs could directly lyse the target cells. With these properties, the obtained CIKs were clearly similar to CIKs derived from fresh UCB samples. In fact, Introna et al.,2006reported that fresh UCB-derived CIKs showed strong cytotoxic activity against a variety of target tumor cell lines. In addition, Li et al., 2010showed that all expanded CB-derived CIKs/NK cells showed cytotoxic activity against the K562 cell line.
Conclusion
UCB has been considered to be a source of HSCs for a long time. However, application of UCB samples to treatment of hematopoietic diseases is limited. The results of the present study suggest that banked UCB samples can act as sources of immune cells, including DCs and CIKs, for immunotherapy. In this study, functional DCs and CIKS were successfully produced from banked UCB samples. The DCs strongly expressed the specific markers of DCs, such as CD40, CD80, CD86, and HLA-DR, phagocytosed an antigen, and efficiently stimulated T cell proliferation. Furthermore, the CIKs strongly inhibited cancer cell proliferation. The present results suggest that banked UCB samples are useful for not only regenerative medicine, but also immunotherapy for cancer treatment.
Abbreviations
CD Cluster of Differentiation; CIK Cytokine-induced killer; DC Dendritic cells; HSC Hematopoietic stem cell; MNC Mononuclear cell; UCB Umbilical cord blood
References
-
F.
Bracho,
C.
Van De Ven,
E.
Areman,
R.
Hughes,
V.
Davenport,
M.
Bradley,
J.
Cai,
M.
Cairo.
A comparison of ex vivo expanded DCs derived from cord blood and mobilized adult peripheral blood plastic-adherent mononuclear cells: decreased alloreactivity of cord blood DCs 1. Cytotherapy.
2003;
5
:
349-361
.
-
J.H.
Dalle,
J.
Menezes,
E.
Wagner,
M.
Blagdon,
J.
Champagne,
M.A.
Champagne,
M.
Duval.
Characterization of cord blood natural killer cells: implications for transplantation and neonatal infections. Pediatr Res.
2005;
57
:
649-655
.
-
L.
Durrieu,
M.M.
Dieng,
F.
Le Deist,
E.
Haddad.
Cord blood-derived and peripheral blood-derived cytokine-induced killer cells are sensitive to Fas-mediated apoptosis. Biol Blood Marrow Transplant.
2013;
19
:
1407-1411
.
-
M.
Enomoto,
H.
Nagayama,
K.
Sato,
Y.
Xu,
S.
Asano,
T.
Takahashi.
In vitro generation of dendritic cells derived from cryopreserved CD34+ cells mobilized into peripheral blood in lymphoma patients. Cytotherapy.
2000;
2
:
95-104
.
-
Y.Y.
Fan,
B.Y.
Yang,
C.Y.
Wu.
Phenotypic and functional heterogeneity of natural killer cells from umbilical cord blood mononuclear cells. Immunol Invest.
2008;
37
:
79-96
.
-
E.
Gluckman.
History of cord blood transplantation. Bone Marrow Transplant.
2009;
44
:
621-626
.
-
E.
Gluckman,
H.A.
Broxmeyer,
A.D.
Auerbach,
H.S.
Friedman,
G.W.
Douglas,
A.
Devergie,
H.
Esperou,
D.
Thierry,
G.
Socie,
P.
Lehn.
Hematopoietic reconstitution in a patient with Fanconi's anemia by means of umbilical-cord blood from an HLA-identical sibling. N Engl J Med.
1989;
321
:
1174-1178
.
-
P.J.
Hanley,
C.R.
Cruz,
E.J.
Shpall,
C.M.
Bollard.
Improving clinical outcomes using adoptively transferred immune cells from umbilical cord blood. Cytotherapy.
2010;
12
:
713-720
.
-
M.
Introna,
M.
Franceschetti,
A.
Ciocca,
G.
Borleri,
E.
Conti,
J.
Golay,
A.
Rambaldi.
Rapid and massive expansion of cord bloodderived cytokine-induced killer cells: an innovative proposal for the treatment of leukemia relapse after cord blood transplantation. Bone marrow transplantation.
2006;
38
:
621-627
.
-
S.K.
Kim,
C.H.
Yun,
S.H.
Han.
Dendritic Cells Differentiated from Human Umbilical Cord Blood-Derived Monocytes Exhibit Tolerogenic Characteristics. Stem Cells Dev.
2015
.
-
J.
Kurtzberg,
M.
Laughlin,
M.L.
Graham,
C.
Smith,
J.F.
Olson,
E.C.
Halperin,
G.
Ciocci,
C.
Carrier,
C.E.
Stevens,
P.
Rubinstein.
Placental blood as a source of hematopoietic stem cells for transplantation into unrelated recipients. N Engl J Med.
1996;
335
:
157-166
.
-
Y.
Li,
I.G.
Schmidt-Wolf,
Y.-F.
Wu,
S.-L.
Huang,
J.
Wei,
J.
Fang,
K.
Huang,
D.-H.
Zhou.
Optimized protocols for generation of cord blood-derived cytokine-induced killer/natural killer cells. Anticancer research.
2010;
30
:
3493-3499
.
-
Y.
Liu,
X.
Tian,
S.
Jiang,
X.
Ren,
F.
Liu,
J.
Yang,
Y.
Chen,
Y.
Jiang.
Umbilical cord blood-derived dendritic cells infected by adenovirus for SP17 expression induce antigen-specific cytotoxic T cells against NSCLC cells. Cell Immunol.
2015
.
-
Q.
Niu,
W.
Wang,
Y.
Li,
S.
Qin,
Y.
Wang,
G.
Wan,
J.
Guan,
W.
Zhu.
Cord blood-derived cytokine-induced killer cells biotherapy combined with second-line chemotherapy in the treatment of advanced solid malignancies. Int Immunopharmacol.
2011;
11
:
449-456
.
-
Y.S.
Park,
C.
Shin,
H.S.
Hwang,
M.
Zenke,
D.W.
Han,
Y.S.
Kang,
K.
Ko,
Y.
Do,
K.
Ko.
In vitro generation of functional dendritic cells differentiated from CD34 negative cells isolated from human umbilical cord blood. Cell Biol Int.
2015;
39
:
1080-1086
.
-
P.V.
Phuc,
V.B.
Ngoc,
D.H.
Lam,
N.T.
Tam,
P.Q.
Viet,
P.K.
Ngoc.
Isolation of three important types of stem cells from the same samples of banked umbilical cord blood. Cell Tissue Bank.
2012;
13
:
341-351
.
-
P.V.
Phuc,
T.H.
Nhung,
D.T.
Loan,
D.C.
Chung,
P.K.
Ngoc.
Differentiating of banked human umbilical cord blood-derived mesenchymal stem cells into insulin-secreting cells. In Vitro Cell Dev Biol Anim.
2011;
47
:
54-63
.
-
L.
Ruggeri,
A.
Mancusi,
M.
Capanni,
E.
Urbani,
A.
Carotti,
T.
Aloisi,
M.
Stern,
D.
Pende,
K.
Perruccio,
E.
Burchielli.
Donor natural killer cell allorecognition of missing self in haploidentical hematopoietic transplantation for acute myeloid leukemia: challenging its predictive value. Blood.
2007;
110
:
433-440
.
-
M.R.
Verneris,
J.S.
Miller.
The phenotypic and functional characteristics of umbilical cord blood and peripheral blood natural killer cells. Br J Haematol.
2009;
147
:
185-191
.
-
L.
Wang,
S.
Huang,
Y.
Dang,
M.
Li,
W.
Bai,
Z.
Zhong,
H.
Zhao,
Y.
Li,
Y.
Liu,
M.
Wu.
Cord blood-derived cytokineinduced killer cellular therapy plus radiation therapy for esophageal cancer: a case report. Medicine (Baltimore).
2014;
93
:
e340
.
-
Y.
Wang,
H.
Xu,
X.
Zheng,
H.
Wei,
R.
Sun,
Z.
Tian.
High expression of NKG2A/CD94 and low expression of granzyme B are associated with reduced cord blood NK cell activity. Cell Mol Immunol.
2007;
4
:
377-382
.
-
Q.
Zhang,
L.
Wang,
C.
Luo,
Z.
Shi,
X.
Cheng,
Z.
Zhang,
Y.
Yang,
Y.
Zhang.
Phenotypic and functional characterization of cytokine-induced killer cells derived from preterm and term infant cord blood. Oncol Rep.
2014;
32
:
2244-2252
.
Comments
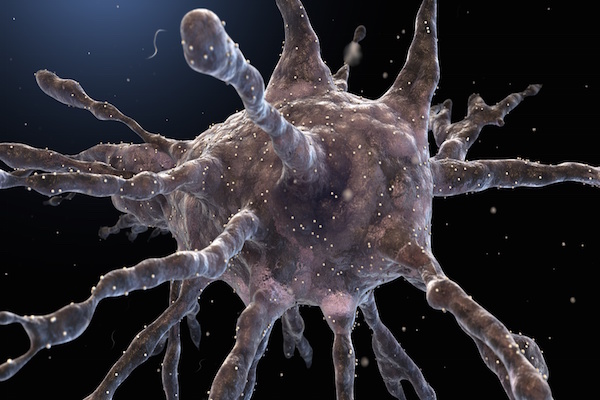
Downloads
Article Details
Volume & Issue : Vol 2 No 11 (2015)
Page No.: 402-408
Published on: 2015-11-30
Citations
Copyrights & License

This work is licensed under a Creative Commons Attribution 4.0 International License.
Search Panel
Pubmed
Google Scholar
Pubmed
Google Scholar
Pubmed
Google Scholar
Pubmed
Google Scholar
Pubmed
Google Scholar
Pubmed
Search for this article in:
Google Scholar
Researchgate
- HTML viewed - 5919 times
- Download PDF downloaded - 1676 times
- View Article downloaded - 4 times