Abstract
Background: Salviamoorcroftiana Wall. ex Benth. is a herbaceous perennial plant indigenous to the Himalayan mountains and especially common in the Kashmir Valley, India. This plant was selected based on its ethnopharmacological promise and the dearth of scientific reporting on its biological activity. This study examined the anticancer therapeutic potential of Salviamoorcroftiana Wall. ex Benth. and also sought to demonstrate its induction of apoptosis, autophagy, and oxidative stress in lung and breast cancer cells.
Methods: Soxhlet's extraction technique was used to obtain an ethanolic extract of Salviamoorcroftiana. A 3-(4,5-dimethylthiazol-2-yl)-2,5-diphenyltetrazolium bromide (MTT) assay was used to evaluate the effects of an ethanolic extract on cell proliferation, and acridine orange/ethidium bromide (AO/EB) staining was used to assess apoptotic cell morphology. Transmission electron microscopy was used to detect autophagy, and 2'-7'dichlorofluorescin diacetate (DCFH-DA) staining was used to measure oxidative stress. Apoptosis and autophagyrelated protein levels were assessed with western blotting.
Results: The ethanolic extract showed concentration and time-dependent inhibition of proliferation against A549 and MDA-MB-231 cell lines with IC50 values of 129.32 µg/ml and 118.12 µg/ml, respectively (*p < 0.05). The AO/EB staining revealed that ethanolic extract brings about apoptotic transformations in both cell lines, further supported by western blotting showing higher caspase 3 and 9 and Bax expression and lower Bcl-2 expression. TEM analysis and western blotting showed the formation of autophagosomes and higher expression of LC3B-I and LC3B-II in A549 and MDA-MB-231, respectively, confirming autophagy. Finally, the DCFH-DA staining showed concentration-reliant augmentation of intracellular ROS production in A549 and MDA-MB-231 cells, indicating the induction of oxidative stress by the ethanolic extract.
Conclusion: This study showed the remarkable anticancer activity of an ethanolic extract of Salvia moorcroftiana Wall. ex Benth. roots via apoptosis, autophagy, and oxidative stress. Natural drug discovery and design against lung and breast cancer could benefit from these findings.
Introduction
Cancer is the most dangerous health condition mankind faces and currently predominates over other health conditions in mortality and global occurrence1. Lung and breast cancer are now becoming common. In the United States, lung cancer is ranked second-most-frequent and is one of the foremost causes of cancer-related death2. More than 0.25 million cases of lung cancer were expected in the U.S. in 116,930 women and 130,340 men3. Previous studies have cited lung cancer as the leading cause of cancer-related deaths in men over 40 years and women over 60 years of age, more than prostate cancer, breast cancer, and leukemia. However, recent years have seen a gradual decrease in lung cancer incidence and mortality because of enhanced tools and mass awareness of screening for the disease. The main risk factor for lung cancer is tobacco consumption via smoking4. The decline in tobacco consumption has reduced the incidence of lung cancer by 48% among men and 23% among women. Secondhand smoke is another leading risk factor contributing to this fatal disease5, 6. These statistics indicate a pressing need for drugs or chemotherapeutics with efficient action against lung cancer.
Breast cancer is another form of harmful neoplasm mostly found in women. Breast cancer is metastatic and invades distant sites, harming other parts of the body as well7. Each year, about 1 million cases of breast cancer are recorded worldwide8. In the United States, breast cancer is the most frequent cancer diagnosed in women, with an incidence rate of 12.5%. The lethality of this illness can be seen in the high incidence of death among patients, 1 in 35 women diagnosed9. Hormone therapy and immune therapies have proven fruitful in eliminating this fatal disease. However, disease recurrence and low survival rates still make this disease a notable challenge to humanity10. The high incidence and death rates of this fatal disease indicate the need for novel management therapies and therapeutics that could eliminate the disease, stop recurrence, and improve survival.
Both lung and breast cancer are lethal health conditions associated with enormous mortality and morbidity globally. Recent advancements in cancer treatment have eased the global cancer burden somewhat, but lung and breast cancers remain hazards that must be addressed by scientists and researchers. Considering the immediate demand for novel treatment options, this study was designed to evaluate the anticancer effects of an ethanolic extract of Salvia moorcroftiana wall. ex Benth. roots. Moreover, the underlying action mechanism was also studied by analyzing apoptosis, autophagy, and oxidative stress.
The Salvia genus belongs to the family Lamiaceae and comprises more than 900 species11. This genus is distributed throughout the world and the most suitable growing conditions are temperate and tropical regions12. Salvia species are important in traditional medicines used worldwide, showing anticancer, antidiabetic, antioxidant, and antibacterial activities13, 14, 15, 16. Salvia moorcroftiana wall. ex Benth. is commonly known as “kallijari” and is native to the Kashmir Valley in the Himalayas. The perennial plant is herbaceous and grows up to 90 cm, with sturdy stems. The roots and seeds are used to treat cough and hemorrhoids and are also used as an emetic. The leaves are used to treat boils and as medicine for itching and guinea worm17. The roots show rich phytochemistry and bioactive phytochemicals18. Despite their rich phytochemistry, very little is known about the pharmacological and medicinal activities of Salvia moorcroftiana wall. ex Benth. roots. This study could help determine the anticancer potential of an ethanolic extract of Salvia moorcroftiana wall. ex Benth. roots against lung and breast cancer cells.
Methods
Chemicals and reagents
RMPI-1640 medium and dichlorodihydrofluorescein diacetate (DCFH-DA) were obtained from Thermo Fisher Scientific, Inc., Waltham, MA, United States. Dimethyl sulphoxide (DMSO), 10% fetal bovine serum, penicillin, streptomycin, 3-(4,5-dimethylthiazol-2-yl)-2,5-diphenyl tetrazolium bromide (MTT), acridine orange/ethidium bromide (AO/EB), phosphate buffer saline (PBS), glutaraldehyde, ethanol, osmium tetroxide, acetone, uranyl acetate, lead citrate, and RIPA lysis buffer were obtained from Sigma-Aldrich; Merck KGaA, Darmstadt, Germany.
Plant material
Salvia moorcroftiana Wall. ex Benth. plant material was collected from hilly areas of District Budgam, Jammu and Kashmir on June 20, 2020. The plant was identified and characterized by Dr. Kanchan Yadav, Department of Botany, Madhyanchal Professional University, Ratibad, Bhopal, Madhya Pradesh; a sample was deposited with her under voucher no. MPU-B-107. The roots of the plant were carefully removed, washed under tap water, and shade dried at 30 – 33 oC for three weeks. The dried roots were powdered with a mortar and pestle. To maintain the quality of the extract, all the dirt and moisture were eliminated. The powder was stored in air-tight plastic bags until extraction.
Ethanolic extraction
The 200 g of powdered, shade-dried Salvia moorcroftiana Wall. ex Benth. roots were extracted with boiling (79 oC) ethanol for 24 h using Soxhlet’s apparatus. The crude extract was concentrated and leftover moisture was eliminated under reduced pressure with a rotatory evaporator. The extract was then stored at 4 oC in a refrigerator. The refrigeration preserved the quality of the extract for future use. Ethanolic extracts of Salvia moorcroftiana Wall. ex Benth. roots were prepared at 12.5, 25, 50, 100, 200, and 400 µg/ml with 0.05% of dimethyl sulphoxide (DMSO) for anticancer analysis.
Cell culture and conditions
The A549 lung cancer cells and MDA-MB-231 breast cancer cells were procured from the National Center for Cell Sciences, Pune, Maharashtra. Both cell lines were maintained in RMPI-1640 medium containing 10% fetal bovine serum, penicillin, and streptomycin. The cell cultures were preserved in a humid environment at 37 oC with 5% CO2.
MTT assay
The MTT was conducted to evaluate the antiproliferative effects of the ethanolic extract of Salvia moorcroftiana Wall. ex Benth. roots against A549 lung and MDA-MB-231 breast cancer cell lines. The A549 and MDA-MB-231 cells were seeded to individual 96-well plates, maintaining a density of 1×105 cells/well. The cultured cell lines were divided into experimental groups, each of which received a different concentration of the ethanolic extract: 0, 12.5, 25, 50, 100, 200, and 400 µg/ml. These treated cell lines were incubated for 24 h, 48 h, and 72 h at 37 oC. After the incubation time, each experimental group received 100 µl of MTT reagent (5 µg/ml) followed by 4 h additional incubation. The formazan crystals formed by the MTT with living cells were dissolved in DMSO and subjected to colorimetric analysis. The optical density of each experimental group was determined by recording the absorbance at 490 nm with a microplate reader. The procedures for each experimental group were performed in triplicate.
AO/EB staining assay
The morphological characteristics of ethanolic extract-treated A549 and MDA-MB-231 cells were monitored by AO/EB staining with fluorescence microscopy. The A549 and MDA-MB-231 cells were seeded in 24-well plates at a density of 1×104 cells/well for 12 h. The cells were divided into experimental groups, each of which received a different concentration of the ethanolic extract: 0, 50, 100, and 200 µg/ml, followed by 24 h of incubation at 37 oC. Afterward, the treated cells were washed twice in PBS and then stained with 20 µl AO/EB staining solution. The stained cells were incubated without light for 15 min. Finally, the morphological and apoptosis characteristics of the treated A549 and MDA-MB-231 cells were assessed under a fluorescent microscope (Nikon Corporation., Tokyo, Japan).
Transmission electron microscopy (TEM)
The autophagy-promoting effects of the ethanolic extract of Salvia moorcroftiana Wall. ex Benth. roots in A549 lung and MDA-MB-231 breast cancer cells were evaluated with TEM analysis. The cell lines were loaded in individual 24-well plates at a concentration of 1×104 cells/well and cultured for 24 h. The cultures were then divided into experimental groups and received either 0 or 100 µg/ml of the extract. Afterward, the cells were incubated for 24 h and then washed and fixed with PBS and glutaraldehyde (2.5%). The washed cells were then stained with 2% osmium tetroxide and subsequently incubated for 2 h at room temperature. The treated and stained A549 and MDA-MB-231 cells were dehydrated with ethanol and then immersed in pure acetone. Before sectioning (5 – 7 µm) under the Ultracut E ultra-thin slicer, the cells were placed in an epoxy resin mixture. The ultrathin sections were stained with lead citrate and uranyl acetate (3%) and then incubated for 20 min without light at room temperature. Finally, the ultrathin stained sections were washed twice in distilled water and subsequently loaded into a transmission electron microscope (Philips Medical Systems, Eindhoven, Netherland).
Reactive oxygen species assessment
The ROS production in treated and control A549 and MDA-MB-231 cells was measured with a DCFH-DA staining assay. The A549 and MDA-MB-231 cells were plated in 24-well plates at a density of 1 × 104 cells/well for 12 h. The cells were divided into experimental groups, each of which received a different concentration of the ethanolic extract: 0, 50, 100, and 200 µg/ml, followed by 24 h of incubation at 37 oC. Afterward, the cells were incubated with 10 mM of DCFH-DA at 37 oC for 25 min. In the presence of intracellular ROS, non-fluorescent DCFH oxidizes to fluorescent DCF. This change was detected by taking absorbance measurements with a fluorescent microplate reader at 570 nm.
Western blotting
The expression of apoptosis and autophagy-related proteins in A549 and MDA-MB-231 cells treated with an ethanolic extract of Salvia moorcroftiana Wall. ex Benth. roots was monitored with western blotting. The A549 and MDA-MB-231 cells were treated with different concentrations of the ethanolic extract (0, 50, 100, and 200 µg/ml) for 24 h. The treated cells were lysed with RIPA lysis buffer. The protein content within each lysate of A549 and MDA-MB-231 cells was measured through a bicinchoninic acid assay. From each sample, about 40 µg of proteins were separated with 10–15% SDS-PAGE and transferred to nitrocellulose membranes blocked with skim milk (5%) for 1 h at room temperature. These membranes were incubated overnight at 4 oC with primary antibodies for caspase-3, caspase-9, Bax, Bcl-2, LC3B-I, and LC3B-II (1:500 dilutions; ThermoFisher Scientific). After primary antibody treatment, the membranes were exposed to HRP conjugated goat anti-rabbit secondary antibodies (1:2,000 dilutions; OriGene Technologies, Inc., Beijing, China) for 1 h without light at 37 oC. Finally, the protein bands were developed with an ECL substrate spray and pictured with the Chemidoc XRS system (Bio-Rad Laboratories, Inc., Hercules, CA, United States).
Statistical analysis
The experimental data of the three triplicate experiments are represented as mean ± SD. The differences among groups were analyzed with a one-way ANOVA followed by Tukey’s test using GraphPad Prism software version 5.0 (GraphPad Software, Inc., La Jolla, CA, United States). A *p < 0.05 was considered statistically significant.
Results
Proliferation inhibition by Salvia moorcroftiana Wall. ex Benth. root extract
The proliferation of A549 and MDA-MB-231 cells was assessed with an MTT assay after treatment with ethanolic extract of Salvia moorcroftiana Wall. ex Benth. roots. The ethanolic extract inhibited the proliferation of A549 cells significantly (p < 0.05). The proliferation inhibition was not only concentration-dependent but time-dependent as well (Figure 1). The proliferation of A549 cells reduced significantly from 100% in controls to almost 23%, 14%, and 7% at 400 µg/ml after 24 h, 48h, and 72 h, respectively. Similar results were observed in the MDA-MB-231 cells, where the cell proliferation was significantly reduced with increasing extract concentration and exposure time. The proliferation reduced from 98% in controls to 18%, 12%, and 9% at 400 µg/ml after 24 h, 48 h, and 72 h, respectively (Figure 2). The ethanolic extract of Salvia moorcroftiana Wall. ex Benth. roots showed an efficient IC50 concentration of 118.12 µg/ml against MDA-MB-231 cells and 129.32 µg/ml against A549 cells.
Apoptosis induction by Salvia moorcroftiana Wall. ex Benth. root extract
Apoptosis in A549 and MDA-MB-231 cells treated with an ethanolic extract of Salvia moorcroftiana Wall. ex Benth. roots was investigated with AO/EB staining and western blotting. The treated A549 cells showed characteristic apoptotic features such as membrane damage, membrane blebbing, formation of apoptotic bodies, nuclear disintegration, DNA damage, and loss of cell integration (Figure 3). The control group showed no such alterations and the overall cell morphology remained normal. The treated MDA-MB-231 cells showed similar morphological changes under fluorescence microscopy. The cell destructive effects of the ethanolic extract were enhanced with increased concentration (0–200 µg/ml). The morphological changes observed after extract treatment are hallmarks of apoptosis and therefore indicate the proapoptotic proliferation inhibition of A549 cells. The proapoptotic antiproliferative effects of the ethanolic extract were further confirmed at the molecular level by evaluating the expression of apoptosis-related proteins in both the A549 and MDA-MB-231 cancer cell lines. The expression of caspase-3, caspase-9, and Bax proapoptotic proteins notably increased with increasing concentrations of ethanolic extract in both target cell lines (Figure 4). Simultaneously, the expression of Bcl-2 antiapoptotic protein decreased dramatically in treated cells compared with the controls. These results suggest that the antiproliferative effects of an ethanolic extract of Salvia moorcroftiana Wall. ex Benth. roots induce apoptosis in both A549 and MDA-MB-231 cells.
Autophagy induction by Salvia moorcroftiana Wall. ex Benth. root extract
Autophagy in A549 and MDA-MB-231 cells treated with an ethanolic extract of Salvia moorcroftiana Wall. ex Benth. roots was monitored with TEM analysis and western blotting. The treated A549 cells showed the formation of autophagosomes under TEM (Figure 5 A). Autophagosomes are hallmarks of autophagic cell death. Autophagy in A549 cells was further confirmed when higher expressions of pro-autophagy proteins LC3B-I and LC3B-II were recorded in treated cells compared with control cells. Similarly, autophagosomes and higher expressions of LC3B-I and LC3B-II were detected in treated MDA-MB-231 cells (Figure 5 B). These results suggest that the ethanolic extract of Salvia moorcroftiana Wall. ex Benth. roots stimulates autophagy in both A549 and MDA-MB-231 cells.
Oxidative stress induced by Salvia moorcroftiana Wall. ex Benth. root extract
The induction of oxidative stress in A549 and MDA-MB-231 cells with an ethanolic extract of Salvia moorcroftiana Wall. ex Benth. roots was assessed with DCFH-DA staining. The results indicate that ROS production in A549 cells was dramatically enhanced after exposure to the ethanolic extract. ROS production increased from 11% in controls to 27%, 45%, 65%, and 85% at 12.5, 50, 100, and 200 µg/ml of ethanolic extract, respectively (Figure 6). Similar results were demonstrated in the MDA-MB-231 cells, where the ROS production increased from 7% to 73% with increasing concentrations (0 – 200 µg/ml) of ethanolic extract (Figure 7). Therefore, the results from DCFH-DA staining indicated that the ethanolic extract of Salvia moorcroftiana Wall. ex Benth. roots induced oxidative stress in both A549 and MDA-MB-231 cells.
Discussion
Medicinal plants are the major source of drugs in the present-day pharmaceutical industry. Due to enormous diversity in the occurrence and structural variety of their phytochemical constituents, medicinal plants have been a source of drug discovery and design since antiquity19. The bioactive phytochemicals identified and isolated from medicinal plants are extensively screened for drugs to treat different human disorders. This led to the discovery of taxol, paclitaxel, vincristine, and vinblastine, natural products that have been incorporated into chemotherapy20. Medicinal plants have been actively used in the pharmaceutical industry.
Salvia species are among the most prevalent medicinal plants with a wide range of therapeutic potential. This genus is a part of Traditional Chinese Medicine, Ayurveda, and other folk medicines worldwide21. Moreover, Salvia species have been the source of numerous bioactive molecules, including cryptotanshinone, castanol C, camphor, and dihydrotanshinone22. Moreover, Salvia species show tremendous anticancer potential against a wide range of human cancers, including prostate, breast, liver, and lung23. Therefore, this study was designed to investigate the anticancer potential of ethanolic extracts of Salvia moorcroftiana Wall. ex Benth. roots and determine its possible mechanism of action by studying apoptosis, autophagy, and oxidative stress in A549 lung and MDA-MB-231 breast cancer cells. The results showed promising antiproliferative effects following a dose- and time-reliant pattern. These results were similar to those of previous studies reporting the antiproliferative effects of Salvia species.
Apoptosis is a natural phenomenon and the proactive form of natural cell death is often termed PCD-I24. Apoptosis is a stepwise process to eliminate damaged, malfunctioning, and cancerous cells to maintain the optimum homeostasis of cells and tissues. Suppression of apoptosis often leads to the pathogenesis of malignant disorders in humans, including cancer. The Bcl-2 family of proteins regulates apoptosis, incorporating both pro-apoptotic (Bax and Bad) and antiapoptotic (Bcl-2) proteins25. Caspases initiate the apoptosis process by regulating the loss of mitochondrial membrane potential and the release of cytochrome c into the cytoplasm, initiating a reaction cascade that ultimately generates apoptosomes or apoptotic bodies26. Triggering apoptosis in cancer cells has become a popular topic for research nowadays because of its natural instigation and selective degradation. Bringing about apoptosis in cancer cells could eliminate the disease at its root. Medicinal plants, including Salvia species, have previously been reported as inducing apoptosis in different human cancer cell lines27, 28. Salvia moorcroftiana tends to upregulate proapoptotic proteins and downregulate antiapoptotic proteins. This study, for the first time, evaluates the proapoptotic effects of an ethanolic extract of Salvia moorcroftiana Wall. ex Benth. roots. We observed that, consistent with previous findings, the ethanolic extract of Salvia moorcroftiana Wall. ex Benth. roots showed proapoptotic effects by inducing nuclear damage, inhibiting Bcl-2, and upregulating caspases and Bax proteins in A549 and MDA-MB-231 cells.
Autophagy is another pivotal survival mechanism that operates based on starvation and stress29. Autophagy is often termed PCD-II and is operated by several factors. The formation of autophagosomes indicates the onset of autophagy. During starvation or fasting, the body's demand for nutrients and energy increases drastically. This is addressed by activating autophagy30. However, this is a self-destructive process in which the body eats its own damaged, malfunctioning, and cancerous cells to manage its nutrient demands during starvation. Established chemotherapeutics target cancer cells, inhibiting the disease by stimulating the autophagy of malignant cells. Many medicinal plants, including Salvia species, have been reported to induce autophagic cell death in cancer cells, but no such evidence had been reported for Salvia moorcroftiana Wall. ex Benth. roots31. This study, for the first time, evaluated the proautophagic potential of an ethanolic extract of Salvia moorcroftiana Wall. ex Benth. roots and found that it induced autophagy in A549 and MDA-MB-231 cells, a finding that was further supported by the overexpression of LC3B-I and LC3B-II.
Oxidative stress is induced by the overproduction of ROS in cells. ROS generation is often associated with damage to genetic material and the pathogenesis of several malignant disorders, including cancer32. ROS are likely to react with almost everything they interact with and often disturb normal cellular processes. Thus, ROS are dangerous to normal cells but can stimulate cell death mechanisms such as autophagy and apoptosis in cancer cells33. This study evaluated the ROS production in A549 and MDA-MB-231 cells after treatment with ethanolic extract and found promising results. The extract induced concentration-reliant oxidative stress in both target cell lines.
Conclusions
In conclusion, this study’s findings revealed that ethanolic extracts of Salvia moorcroftiana Wall. ex Benth. roots possess remarkable anticancer potency against the A549 lung and MDA-MB-231 breast cancer cell lines. Furthermore, the ethanolic extract induced apoptosis, autophagy, and oxidative stress in A549 and MDA-MB-231 cells. Therefore, our study offers a foundation for identifying and isolating bioactive entities in the extract that can contribute to lung and breast cancer research and treatment.
Abbreviations
AO/EB: Acridine Orange/Ethidium Bromide
Bad: Bcl-2 associated agonist of cell death
Bax: Bcl-2-associated X protein
Bcl-2: B-cell lymphoma 2
DCFH-DA: Dichloro-dihydro-fluorescein diacetate
PCD: Programmed Cell Death
ROS: Reactive oxygen species
TEM: Transmission electron microscopy
Acknowledgments
Authors are highly thankful to the Department of Pharmacy and Department of Chemistry at Madhyanchal Professional University, Ratibad, Bhopal-462044, M.P, INDIA, for providing academic support and necessary equipment’s.
Author’s contributions
All the authors contributed equally in designing, execution, data interpretation and writing of this research work. All authors read and approved the final manuscript.
Funding
None.
Availability of data and materials
Data and materials used and/or analyzed during the current study are available from the corresponding author on reasonable request.
Ethics approval and consent to participate
Not applicable.
Consent for publication
Not applicable.
Competing interests
The authors declare that they have no competing interests.
References
-
Fitzmaurice
C.,
Dicker
D.,
Pain
A.,
Hamavid
H.,
Moradi-Lakeh
M.,
MacIntyre
M.F.,
Global Burden of Disease Cancer Collaboration
The global burden of cancer 2013. JAMA Oncology.
2015;
1
(4)
:
505-27
.
View Article PubMed Google Scholar -
Alexander
M.,
Kim
S.Y.,
Cheng
H.,
Update 2020: Management of Non-Small Cell Lung Cancer. Lung.
2020;
198
(6)
:
897-907
.
View Article PubMed Google Scholar -
Siegel
R.L.,
Miller
K.D.,
Jemal
A.,
Cancer statistics, 2020. CA: a Cancer Journal for Clinicians.
2020;
70
(1)
:
7-30
.
View Article PubMed Google Scholar -
Jeon
J.,
Holford
T.R.,
Levy
D.T.,
Feuer
E.J.,
Cao
P.,
Tam
J.,
Smoking and lung cancer mortality in the United States from 2015 to 2065: a comparative modeling approach. Annals of Internal Medicine.
2018;
169
(10)
:
684-93
.
View Article PubMed Google Scholar -
Couraud
S.,
Zalcman
G.,
Milleron
B.,
Morin
F.,
Souquet
P.J.,
Lung cancer in never smokers: review. European Journal of Cancer (Oxford, England).
2012;
48
(9)
:
1299-311
.
View Article PubMed Google Scholar -
Kim
A.S.,
Ko
H.J.,
Kwon
J.H.,
Lee
J.M.,
Exposure to secondhand smoke and risk of cancer in never smokers: a meta-analysis of epidemiologic studies. International Journal of Environmental Research and Public Health.
2018;
15
(9)
:
1981
.
View Article PubMed Google Scholar -
Scully
O.J.,
Bay
B.H.,
Yip
G.,
Yu
Y.,
Breast cancer metastasis. Cancer Genomics & Proteomics.
2012;
9
(5)
:
311-20
.
PubMed Google Scholar -
McPherson
K.,
Steel
C.M.,
Dixon
J.M.,
ABC of breast diseases. Breast cancer-epidemiology, risk factors, and genetics. BMJ (Clinical Research Ed.).
2000;
321
(7261)
:
624-8
.
View Article PubMed Google Scholar -
Lynch
H.T.,
Watson
P.,
Conway
T.A.,
Lynch
J.F.,
Clinical/genetic features in hereditary breast cancer. Breast Cancer Research and Treatment.
1990;
15
(2)
:
63-71
.
View Article PubMed Google Scholar -
Bombonati
A.,
Sgroi
D.C.,
The molecular pathology of breast cancer progression. The Journal of Pathology.
2011;
223
(2)
:
307-17
.
View Article PubMed Google Scholar -
Walker
J.B.,
Sytsma
K.J.,
Treutlein
J.,
Wink
M.,
Salvia (Lamiaceae) is not monophyletic: implications for the systematics, radiation, and ecological specializations of Salvia and tribe Mentheae. American Journal of Botany.
2004;
91
(7)
:
1115-25
.
View Article PubMed Google Scholar -
Min-hui
L.,
Jian-min
C.,
Yong
P.,
Pei-gen
X.,
Distribution of phenolic acids in Chinese Salvia plants. Wood Science and Technology.
2008;
10
(5)
:
46-52
.
-
Privitera
G.,
Luca
T.,
Castorina
S.,
Passanisi
R.,
Ruberto
G.,
Napoli
E.,
Anticancer activity of Salvia officinalis essential oil and its principal constituents against hormone-dependent tumour cells. Asian Pacific Journal of Tropical Biomedicine.
2019;
9
(1)
:
24
.
View Article Google Scholar -
Vergine
M.,
Nicoli
F.,
Negro
C.,
Luvisi
A.,
Nutricati
E.,
Accogli
R.A.,
Phytochemical profiles and antioxidant activity of Salvia species from Southern Italy. Records of Natural Products.
2019;
13
(3)
:
215
.
View Article Google Scholar -
Mahdizadeh
R.,
Moein
S.,
Soltani
N.,
Malekzadeh
K.,
Mahmoodreza
M.,
Study the molecular mechanism of salvia species in prevention of diabete. International Journal Of Pharmaceutical Sciences And Research.
2018;
9
(11)
:
4512-21
.
-
Delamare
A.P.,
Moschen-Pistorello
I.T.,
Artico
L.,
Atti-Serafini
L.,
Echeverrigaray
S.,
Antibacterial activity of the essential oils of Salvia officinalis L. and Salvia triloba L. cultivated in South Brazil. Food Chemistry.
2007;
100
(2)
:
603-8
.
View Article Google Scholar -
Rather
M.A.,
Dar
B.A.,
Bhat
K.A.,
Shawl
A.S.,
Qurishi
M.A.,
Dar
M.Y.,
Mono-sesquiterpenoid composition in the leaves and flowers of Salvia moorcroftiana Wall ex Benth. growing wild in Kashmir, India. The Journal of Essential Oil Research.
2011;
23
(4)
:
21-5
.
View Article Google Scholar -
Kabouche
A.,
Boutaghane
N.,
Kabouche
Z.,
Seguin
E.,
Tillequin
F.,
Benlabed
K.,
Components and antibacterial activity of the roots of Salvia jaminiana. Fitoterapia.
2005;
76
(5)
:
450-2
.
View Article PubMed Google Scholar -
Khan
T.,
Ali
M.,
Khan
A.,
Nisar
P.,
Jan
S.A.,
Afridi
S.,
Anticancer plants: A review of the active phytochemicals, applications in animal models, and regulatory aspects. Biomolecules.
2019;
10
(1)
:
47
.
View Article PubMed Google Scholar -
Gezici
S.,
Sekeroglu
N.,
Current Perspectives in the Application of Medicinal Plants Against Cancer: Novel Therapeutic Agents . Anticancer Agents Med Chem.
2019;
19
(1)
:
101-111
.
View Article PubMed Google Scholar -
Fan
T.P.,
Zhu
Y.,
Leon
C.,
Franz
G.,
Bender
A.,
Zheng
X.,
Traditional Chinese Medicine Herbal Drugs: From Heritage to Future Developments. In The Science and Regulations of Naturally Derived Complex DrugsSpringer: Cham; 2019.
View Article Google Scholar -
Jiang
Y.,
Zhang
L.,
Rupasinghe
H.V.,
The anticancer properties of phytochemical extracts from Salvia plants. Botanics : Targets and Therapy.
2016;
6
:
25-44
.
-
Hao
D.C.,
Ge
G.B.,
Xiao
P.G.,
Anticancer drug targets of Salvia phytometabolites: chemistry, Biology and omics. Current Drug Targets.
2018;
19
(1)
:
1-20
.
View Article PubMed Google Scholar -
Pfeffer
C.M.,
Singh
A.T.,
Apoptosis: a target for anticancer therapy. International Journal of Molecular Sciences.
2018;
19
(2)
:
448
.
View Article PubMed Google Scholar -
Singh
R.,
Letai
A.,
Sarosiek
K.,
Regulation of apoptosis in health and disease: the balancing act of BCL-2 family proteins. Nature Reviews. Molecular Cell Biology.
2019;
20
(3)
:
175-93
.
View Article PubMed Google Scholar -
Cheng
X.,
Ferrell
J.E.,
Apoptosis propagates through the cytoplasm as trigger waves. Science.
2018;
361
(6402)
:
607-12
.
View Article PubMed Google Scholar -
Russo
A.,
Cardile
V.,
Graziano
A.C.,
Avola
R.,
Bruno
M.,
Rigano
D.,
Involvement of Bax and Bcl-2 in induction of apoptosis by essential oils of three Lebanese Salvia species in human prostate cancer cells. International Journal of Molecular Sciences.
2018;
19
(1)
:
292
.
View Article PubMed Google Scholar -
Khursheed
A.,
Rather
M.A.,
Rashid
R.,
Plant-based natural compounds and herbal extracts as promising apoptotic agents: their implications for cancer prevention and treatment. Adv. Biomed. Pharma..
2016;
3
(4)
:
245-69
.
View Article Google Scholar -
Li
X.,
He
S.,
Ma
B.,
Autophagy and autophagy-related proteins in cancer. Molecular Cancer.
2020;
19
(1)
:
12
.
View Article PubMed Google Scholar -
Yun
C.W.,
Lee
S.H.,
The roles of autophagy in cancer. International Journal of Molecular Sciences.
2018;
19
(11)
:
3466
.
View Article PubMed Google Scholar -
Ko
M.,
Oh
G.T.,
Park
J.,
Kwon
H.J.,
Extract of high hydrostatic pressure-treated danshen (Salvia miltiorrhiza) ameliorates atherosclerosis via autophagy induction. BMB Reports.
2020;
53
(12)
:
652-7
.
View Article PubMed Google Scholar -
Klaunig
J.E.,
Wang
Z.,
Oxidative stress in carcinogenesis. Current Opinion in Toxicology.
2018;
7
:
116-21
.
View Article Google Scholar -
Kunchithapautham
K.,
Rohrer
B.,
Apoptosis and autophagy in photoreceptors exposed to oxidative stress. Autophagy.
2007;
3
(5)
:
433-41
.
View Article PubMed Google Scholar
Comments
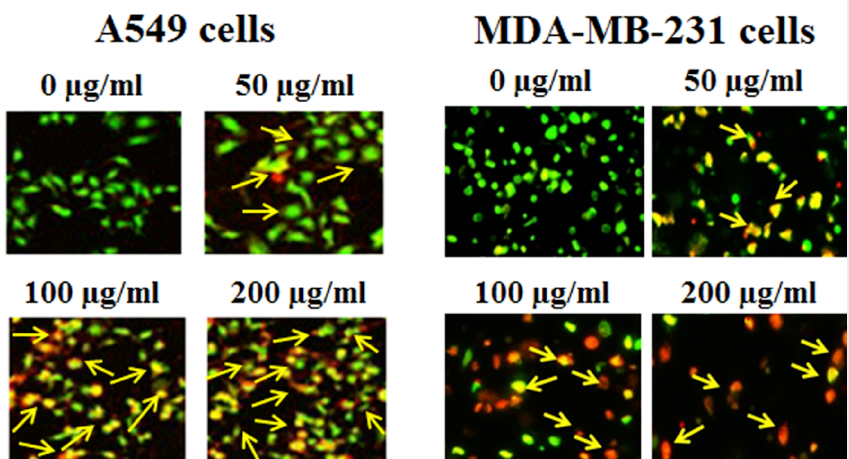
Article Details
Volume & Issue : Vol 8 No 11 (2021)
Page No.: 4718-4727
Published on: 2021-11-30
Citations
Copyrights & License

This work is licensed under a Creative Commons Attribution 4.0 International License.
Search Panel
- HTML viewed - 5279 times
- PDF downloaded - 1505 times
- XML downloaded - 0 times