Abstract
Background: Lateral flow immunoassay (LFIA) is a well-known method because of its extensive application in many fields, such as medical, food safety, and environmental analysis. LFIA is also under development for pathogen or protein detection, where rapid detection is essential. However, there are few studies on the optimal process for LFIA production.
Methods: This study aimed to evaluate the roles of the different materials that make up the LFIA strip, consisting of the sample pad, conjugate pad, nitrocellulose membrane, and absorbent pad. Different membranes and pads were evaluate regarding membrane permeability, generation of the test line, capillary flow time, and result visibility.
Results: The results indicated that the optimized LFIA strip is composed of four membranes and pads, including the CF3 sample pad, FF170HP nitrocellulose membrane immobilized with 1 mg of antibody, Standard 17 conjugate pad, and CF6 absorbent pad. The result was visually detectable within 15 minutes.
Conclusion: This optimization protocol could be a reference for the development of new test strips.
Introduction
The premise behind the lateral flow immunoassay (LFIA) or quick test is the latex agglutination test that was developed in 1956 by Plotz and Singer1. Although there are several variations of this technology, such as the radioimmunoassay (RIA) and enzyme immunoassay (EIA), they all function on the principle of antigen-antibody reaction. LFIA technology continued to develop and was expanded in the pregnancy test market in the 1980s. The LFIA test was the primary test used to detect HCG2. Since then, LFIA tests have been used in various fields, including medical, pharmaceutical, food safety, and environmental analysis. LFIA is a powerful and suitable test when applied to an expanded range of point-of-care (POC) or field applications1 due to its merits of straightforwardness, rate, low-cost, portable devices, ease of use, and no prerequisite of apparatus or specialized skills3, 4, 5.
In general, the LFIA has four elemental constituents comprising a sample pad, a conjugate pad, a nitrocellulose membrane (NCM), and an absorbent (wick) pad. These materials are overlapped at the rear surface and fixed to a backing card. The optimal properties of the pads and NCM are a steady wicking rate and low nonspecific binding1, 5, 6. There are three types of pads, including sample pads, conjugate pads, and absorbent pads, and they are made from porous materials. Cellulosic material is used for the sample and absorbent pads, while glass fiber is used for the conjugate pad5, 6. The sample pad is selected based on the sample type and sample volume. Certain properties of the conjugate pad, including thickness and nonspecific binding, can affect the release of the labeled conjugate and the sensitivity of the assay. NCMs are the key component of LFIAs because of their usefulness, low cost, and high affinity for proteins and biomolecules3, 5, 6, 7. The material characteristics of the NCM, such as thickness and capillary flow rate, influence the sensitivity of the LFIA1, 6.
The principle of LFIA can be described as follows. First, a sample is added to the LFIA strip and enters the sample pad. Second, the sample reaches the conjugate pad, which releases the colloidal gold antibody conjugate, and there is an interaction between the analytes in the sample and the labeled antibody. Then, the sample travels to the NCM, where the target-specific antibody at the test line and the anti-target-specific IgG antibody at the control line are immobilized. The color appearance of both the test and control lines demonstrates a positive result; if only one colored line appears in the control area, the analyte is absent from the sample. Finally, the sample enters the absorbent pad, which provides sufficient bed volume for the total flow of the test (Figure 1). The function of the LFIA depends on capillary forces provided by the absorbent pad to facilitate the movement of the sample along a test strip, and a signal is generated after a brief period. However, it is difficult to establish a general LFIA protocol for all applications because of the target-specific characteristics of the assay. Therefore, the purpose of this study was to describe the basic protocol for optimizing the membrane components of LFIAs on a laboratory scale.
Proteins serve as biomarkers for the detection of several diseases, and the analysis of these proteins plays a key role in clinical diagnosis. Most lateral flow test strips are designed based on antigen-antibody interactions; proteins in the sample react with specific antibodies immobilized on the membrane. Therefore, LFIA can be applied to detect specific targets if specific antibodies to those targets are available. In the present study, the optimization protocol of LFIA for shrimp acute hepatopancreatic necrosis disease (AHPND) was used as an example. AHPND in shrimp is caused by two secretory toxins from Vibrio parahaemolyticus, PirAvp and PirBvp, with approximate molecular weights of 16 kDa and 50 kDa, respectively8. Therefore, two test lines were immobilized with anti-PirAvp and anti-PirBvp polyclonal antibodies in the LFIA. Certain parameters must be considered in the optimization of LFIA: NCMs were evaluated for their ability to produce target lines, absorbent pads were evaluated for their capillary flow time, conjugated pads were evaluated for membrane permeability and the generation of the test line, and sample pads were evaluated for their ability to draw the sample along the test strip.
Methods
Materials
The backing card (MIBA-020-60 mm) and colloidal gold solution (CG-020) were obtained from DCNovations, USA. Membranes and pads were purchased from Cytiva Life Sciences, Sweden. Three types of NCMs were obtained: Whatman FF80HP, FF120HP, and FF170HP, with capillary flow rates of 60–100 s/4 cm, 90–150 s/4 cm, and 140–200 s/4 cm, respectively.
Sample pads included Whatman CF1 and CF3, with thicknesses of 176 µm and 322 µm, respectively, and capillary flow rates of 207.3 s/4 cm and 174.3 s/4 cm, respectively.
Conjugate pads included Fusion 5, Whatman Standard 17, and Standard 14, with thicknesses of 370 µm, 370 µm, and 355 µm, respectively, and capillary flow rates of 38 s/4 cm, 34.5 s/4 cm, and 23.1 s/4 cm, respectively.
Absorbent pads included Whatman CF5, CF6, and CF7, with thicknesses of 594 µm, 1450 µm, and 1873 µm, respectively, and capillary flow rates of 63.3 s/4 cm, 65 s/4 cm, and 35 s/4 cm, respectively.
Other chemicals were of analytical grade.
Optimization of the nitrocellulose membrane
Three types of membranes, including Whatman FF80HP, FF120HP, and FF170HP (Cytiva Life Sciences), were evaluated for membrane permeability and generation of the test line. Antibodies were sprayed onto the membranes using an Automated Lateral Flow Reagent Dispenser (ClaremontBio, USA), and the membranes were cut into 5-mm-wide strips by a programmable strip cutter (Shanghai Kinbio Tech, China). After spraying, the formed antibody lines were assessed for membrane permeability with the naked eye. The types of test strip membranes were then evaluated for their ability to create target lines. The antigen and the colloidal gold-labeled antibody were mixed and applied onto the membranes.
Optimization of the absorbent pad
Whatman CF5, CF6, and CF7 absorbent pads were mounted onto the adhesive plastic backing card that already contained an optimized NCM. The absorbent pad overlapped with the NCM for a length of approximately 3 mm and was cut into 5-mm-wide strips using a programmable strip cutter (Shanghai Kinbio Tech, China).
Optimization of conjugate pad
Fusion 5, Whatman Standard 17, and Standard 14 conjugate pads were mounted onto the adhesive plastic backing card that already contained an optimized NCM and absorbent pad. The conjugate pad overlapped with the NCM for a length of approximately 3 mm and was cut into 5-mm-wide strips using a programmable strip cutter (Shanghai Kinbio Tech, China).
Optimization of the sample pad
Whatman CF1 and CF3 sample pads were mounted onto the adhesive plastic backing card that already contained an optimized NCM, absorbent pad, and conjugate pad. The sample pad overlapped with the conjugate pad for approximately 3 mm of the conjugate pad and was cut into 5-mm-wide strips using a programmable strip cutter (Shanghai Kinbio Tech, China).
Optimization of the amount of antibody immobilized in the test line
After the selection of the optimized membranes and pads, these components were fixed to the adhesive backing card. The parts were placed on one side with 3 mm of overlap with each another. The LFIA was cut into 5-mm-wide strips and placed in a desiccator container at 4 °C. Different amounts of anti-target antibodies (0.5, 1, and 2 µg/strip) were immobilized onto the NCMs. A mixture of the purified target proteins in the running buffer was spiked at 500 ng/ml, and 100 µL was loaded into the test strip. A negative sample of the running buffer was added at the same time to another test strip. The result was observed after 15 minutes with the naked eye. The appearance of red lines at the test line and control line indicated the presence of the analyte in the sample. Only one colored line in the control area indicated the absence of an analyte from the sample.
Statistical analysis
Data were analyzed and compared using GraphPad Prism 7 software. The data from three independent repeats are reported as the mean ± standard deviation (SD) and were compared using one-way analysis of variance (ANOVA). The differences were considered significant if p < 0.05.
Results
Optimization of the nitrocellulose membrane
After spraying, the formed antibody lines were observed. For the FF120HP and FF170HP membranes, the proteins formed continuous lines (Figure 2 A, samples 2 and 3). The FF80HP membrane produced dashed lines with many small dots (Figure 2 A, sample 1) therefore, the colored signal was also generated in dots, with smearing and even a lost signal (Figure 2 B, sample 1). The signals on the FF120HP and FF170HP membranes were concentrated; however, there was smearing on the FF120HP membrane (Figure 2 B, sample 2, lower line). The results demonstrated the relationship between membrane permeability and the generation of the test line. The FF170HP membrane was selected for further study.
Optimization of the absorbent pad
Three types of absorbent pads with different thicknesses were mounted onto the adhesive plastic backing card that already contained an FF170HP membrane and was cut into test strips. During the cutting process, the CF5 and CF6 pads were easier to cut than the CF7 pad (data not shown). Because the CF7 pad was too thick, it was difficult to insert into the cutter and slide on the surface of the device, which caused tearing at the edge of the membrane (Figure 3 A, sample 3). All three pads guided the entire sample volume through the strip. For the CF6 and CF7 pads, the sample was pulled at the same rate, and the time for the entire sample solution to reach the membrane was 8 minutes, while the CF5 pad took 13 minutes (Table 1). Moreover, the CF6 pad generated a regular flow front without lamination defects (Figure 3 B, sample 2), while the CF5 and CF7 pads did not (Figure 3 A, samples 1 and 3). Therefore, the CF6 pad was selected for further study.
Groups | Count | Average | Variance |
---|---|---|---|
CF5 | 3 | 13.500a | 0.250 |
CF6 | 3 | 8.233b | 0.063 |
CF7 | 3 | 8.333c | 0.123 |
Optimization of the conjugate pad
Fusion 5, Whatman Standard 17, and Standard 14 conjugate pads were loaded with the colloidal gold polyclonal antibody conjugate and dried at 37 °C for 15 minutes. After drying, the Standard 17 and Fusion 5 pads were almost completely dry, while the Standard 14 pad was still wet, which affected pad attachment to the test strip (Figure 4).
When the sample was loaded onto the test strip, most of the gold-labeled antibody conjugate migrated completely onto the NCM for the Fusion 5 and Standard 17 pads, while a large amount of sample remained in the conjugate pad for the Standard 14. Moreover, the Standard 14 pad test line was smeared (Figure 4 B, sample 1). Although the Standard 17 and Fusion 5 pads produced the same degree of permeability, the color intensity at the test line of the Fusion 5 pad was weaker than that of the Standard 17 pad (Figure 4 B, samples 2 and 3). Therefore, the Standard 17 pad was selected for further study.
Optimization of the sample pad
Whatman CF1 and CF3 sample pads were mounted onto the adhesive plastic backing card that already contained an FF170HP membrane, CF6 pad, and Standard 17 pad, and they were cut into 5-mm-wide strips. Both pads generated the same capillary force; however, the CF3 pad was chosen for the next step because its thickness facilitated the maintenance of a relatively constant flow rate of solution on the membrane (Figure 5). Moreover, the CF3 pad was more efficient than the CF1 pad due to its thickness. The thicker CF3 pad resulted in a higher buffering capacity and more stable flow5. In addition, the flow rate of the CF3 pad (174.3 s/4 cm) was slower than that of the CF1 pad (207.3 s/4 cm), which increased the sensitivity of the test strip due to the prolonged interaction between the target and the gold-particle conjugate.
Optimization of the amount of antibody immobilized to the test lines
LFIA strips with different amounts of immobilized anti-PirAvp and anti-PirBvp polyclonal antibodies were used to evaluate the test line intensity. The signal intensity was observed to be identical for 1 and 2 µg of antibody but was higher than that of 0.5 µg antibody (Figure 6). Therefore, 1 µg of antibody was chosen to be immobilized onto the NCM, which would be economical while still ensuring the detection of toxins.
In summary, the optimized LFIA was a combination of the CF3 sample pad, the Standard 17 conjugate pad, the FF170HP NCM immobilized with 1 µg of antibody, and the CF6 absorbent pad.
Discussion
Lateral flow immunoassays are composed of predetermined components, including a sample pad, conjugate pad, NCM, and absorbent (wick) pad. To ensure proper operation of the test strip, the assembly must be consistent so that the flow front is regular and uniform in all strips otherwise, a lamination defect can occur. A lamination defect can be caused by material incompatibility, overcompression of the assembly elements, or material characteristics. During the development of the LFIA, the most effort is concentrated on selecting the optimal antibody; however, it is necessary to consider the basic materials that comprise the sample pad, conjugate pad, absorbent pad, and NCM to ensure a high-quality and uniform test strip9. The sample pad is the starting point of the test strip where the sample is loaded; it plays a key role in evenly distributing and directing the sample to the conjugate pad. In addition, it eliminates confounding components from samples3, 5, 6. In this study, the Whatman CF3 pad was more effective than the CF1 pad due to its increased thickness. The thickness of the pad influenced both the bed volume and flow. A thicker pad increases the buffering capacity and stability of the flow rate5; a slower flow increases the sensitivity of the test strip and prolongs the interaction between the analyte and the labeled conjugate.
The NCM is the most important material component because it determines the total flow rate of the system and the time required to obtain a signal7. The physicochemical properties of the membrane influence the capillary flow, which is related to sample migration, sensitivity, specificity, and test line consistency5. The capillary flow rates of NCMs are exceptionally challenging determining precisely as the rate decreases exponentially as the solution passes through the membrane6. Therefore, membrane lateral flow is assessed on the premise of capillary flow time, which is the time required for the solution to permeate and fully cover a 4-cm-long strip of the membrane1, 5, 6. A higher capillary flow rate prolongs the reaction between the toxins and the gold-labeled antibody, increasing the sensitivity of the strip test. However, a high capillary flow rate also increases the nonspecific interaction5. In this study, NCMs with distinctive capillary flow rates, including Whatman FF80HP (60–100 s/4 cm), Whatman FF120HP (90–150 s/4 cm), and Whatman FF170HP (140–200 s/4 cm), were evaluated; Whatman FF170HP generated the highest intensity.
The amount of immobilized antibody in the test and control lines also plays a key role in the interaction between antigen and antibody and influences the generation of sensitive and reproducible assays. A weak signal is caused by a low amount of antibody binding to the membrane7; however, if too much antibody is immobilized onto the membrane, it will be uneconomical and lead to an increase in cost. Therefore, 1 µg of antibody was chosen to be immobilized onto the NCM because it is economical while still ensuring the detection of toxins.
The conjugate pad is where the gold-labeled antibodies are preserved upon drying and released upon rewetting. Preparation of the conjugate pad is one of the critical steps during the manufacturing of the test strip5, 6, 10. After drying, the conjugate pad must release the conjugates sufficiently and stably1.
The absorbent pad is the final material in the LFIA. It acts as a sink to extend the total volume of sample that passes through the strip. The thickness of the absorbent pad provides sufficient bed volume to wash nonspecific components away from the test and control lines, thereby reducing the noise signal and increasing the assay sensitivity5, 6. If the absorbent pad is too thick, it is difficult to insert into the cutter and slide on the surface of the device, which can cause tearing at the edge of the membrane.
The lamination process of the membrane and pads is the final stage in the production of the LFIA. The NCM is fixed onto the adhesive plastic backing card, and the absorbent pad, conjugate pad, and sample pad are placed. These materials must overlap properly to ensure that the sample flow moves homogenously from the sample pad to the absorbent pad and to ensure batch-to-batch reproducibility1, 5.
AHPND is a dangerous emerging disease in shrimp that has caused significant economic losses. Early disease detection is essential to control and treat infected shrimp11. Therefore, test strips were developed in several studies for the rapid detection of the causative pathogens of AHPND. The test detects either PirAvp protein or PirBvp protein; for example, a polyclonal antibody is used to detect PirAvp or a monoclonal antibody is used to detect PirBvp12. Our study used polyclonal antibodies to simultaneously detect both PirAvp and PirBvp proteins, which facilitated rapid screening for AHPND pathogens at the pondsite.
Conclusions
In summary, we developed and optimized an LFIA strip for the rapid simultaneous detection of both PirAvp and PirBvp proteins on a laboratory scale. The LFIA strip was applied for the early detection of AHPND at the pond site. However, the design and optimization of LFIAs remain challenging. Multiple parameters, such as the types of nanoparticles and fluid dispensing, and drying conditions, need to be standardized among all strips to ensure batch-to-batch reproducibility.
Abbreviations
AHPND: acute hepatopancreatic necrosis disease, EIA: enzyme immunoassay, LFIA: lateral flow immunoassay, POC: point-of-care, RIA: radioimmunoassay
Acknowledgments
None.
Author’s contributions
NDD: Conceptualization, Data curation, Formal analysis, Methodology, Validation, Writing – review and editing. KHNP: Conceptualization, Data curation, Formal analysis, Methodology, Validation, Writing - review and editing. KYTD: Data curation, Formal analysis, Methodology, Validation. TDMH, NTTN: Investigation, Methodology. TLT: Conceptualization, Supervision. HTV: Conceptualization, Project administration, Supervision, Writing - review and editing. All authors read and approved the final manuscript.
Funding
None.
Availability of data and materials
Data and materials used and/or analyzed during the current study are available from the corresponding author on reasonable request.
Ethics approval and consent to participate
Not applicable.
Consent for publication
Not applicable.
Competing interests
The authors declare that they have no competing interests.
References
-
Wong
R.,
Tse
H.,
Lateral flow immunoassay. Springer Science & Business Media 2008.
View Article Google Scholar -
Vashist
S.K.,
Luong
J.H.,
Handbook of immunoassay technologies: approaches, performances, and applications. Academic Press 2018.
Google Scholar -
NanoComposix. Lateral Flow Assay Development Guide; 2016. Available from: https://www.stratech.co.uk/wp-content/uploads/2016/10/BioReady-Lateral-Flow-Handbook-v-1.0.pdf..
.
-
Anfossi
L.,
Di Nardo
F.,
Cavalera
S.,
Giovannoli
C.,
Baggiani
C.,
Multiplex lateral flow immunoassay: an overview of strategies towards high-throughput point-of-need testing. Biosensors (Basel).
2018;
9
(1)
:
2
.
View Article PubMed Google Scholar -
Parolo
C.,
Sena-Torralba
A.,
Bergua
J.F.,
Calucho
E.,
Fuentes-Chust
C.,
Hu
L.,
Tutorial: design and fabrication of nanoparticle-based lateral-flow immunoassays. Nature Protocols.
2020;
15
(12)
:
3788-816
.
View Article PubMed Google Scholar -
Millipore
E.M.,
Rapid lateral flow test strips: considerations for product development. EMD Millipore Corporation. Billerica, MA, USA..
2013;
29
:
702-7
.
-
Mansfield
M.A.,
Nitrocellulose membranes for lateral flow immunoassays: a technical treatise. Lateral flow immunoassay.
2009;
:
1-19
.
View Article Google Scholar -
Sirikharin
R.,
Taengchaiyaphum
S.,
Sanguanrut
P.,
Chi
T.D.,
Mavichak
R.,
Proespraiwong
P.,
Characterization and PCR detection of binary, Pir-like toxins from Vibrio parahaemolyticus isolates that cause acute hepatopancreatic necrosis disease (AHPND) in shrimp. PLoS One.
2015;
10
(5)
:
e0126987
.
View Article PubMed Google Scholar -
Koczula
K.M.,
Gallotta
A.,
Lateral flow assays. Essays in Biochemistry.
2016;
60
(1)
:
111-20
.
View Article PubMed Google Scholar -
Innova Biosciences. Guide to Lateral Flow Immunoassays; 2016. Available from: https://fnkprddata.blob.core.windows.net/domestic/download/pdf/IBS_A_guide_to_lateral_flow_immunoassays.pdf. 2016
.
-
Wangman
P.,
Chaivisuthangkura
P.,
Taengchaiyaphum
S.,
Pengsuk
C.,
Sithigorngul
P.,
Longyant
S.,
Development of a rapid immunochromatographic strip test for the detection of Vibrio parahaemolyticus toxin B that cause acute hepatopancreatic necrosis disease. Journal of Fish Diseases.
2020;
43
(2)
:
207-14
.
View Article PubMed Google Scholar -
Kamsagara Hanumanthappa
S.,
Billekallu Thammegowda
N.K.,
Patil
P.,
Rama Poojary
S.,
Purandara Ballyaya
A.,
Kavalagiriyanahalli Srinivasaiah
R.,
Polyclonal antibody-based farmer-friendly flow-through test for the detection of acute hepatopancreatic necrosis disease in shrimp. Aquaculture Research.
2020;
51
(7)
:
2863-9
.
View Article Google Scholar
Comments
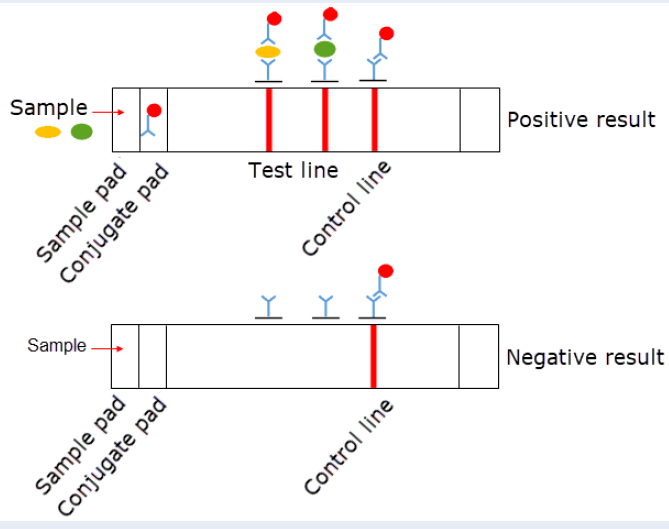
Article Details
Volume & Issue : Vol 10 No 1 (2023)
Page No.: 5500-5508
Published on: 2023-01-31
Citations
Copyrights & License

This work is licensed under a Creative Commons Attribution 4.0 International License.
Search Panel
Pubmed
Google Scholar
Pubmed
Google Scholar
Pubmed
Google Scholar
Pubmed
Google Scholar
Pubmed
Google Scholar
Pubmed
Google Scholar
Pubmed
Search for this article in:
Google Scholar
Researchgate
- HTML viewed - 6906 times
- PDF downloaded - 2345 times
- XML downloaded - 0 times