Adipose derived stem cell transplantation is better than bone marrow mesenchymal stem cell transplantation in treating hindlimb ischemia in mice
Abstract
Introduction
Ischemia is conditionally caused by limited blood supply to tissues, which results in a shortage of oxygen and nutrients needed for cellular activities. The main cause of ischemia is damage to blood vessels. Ischemia can occur at different sites, tissues and organs in the human body and can cause diseases, such as hindlimb ischemia, stroke and myocardial infarction. Hindlimb ischemia, particularly acute hindlimb ischemia (ALI), is a popular model of ischemia studied in rat, mouse and rabbit models for evaluation of suitable therapies.
ALI is a serious condition of peripheral artery disease (PAD). Its incidence is predicted to increase due to the rising rate of diabetes, especially among the elderly population in the United States. Approximately 8-14 million people in the U.S. and about 202 million people worldwide suffered from PAD in 2010; the percentage of patients with classic symptoms of claudication was about 10%; approximately 40% of patients did not complain of pain in the legs and the remaining 50% showed various signs and symptoms Mozaffarian et al.,2016. During the last decade, PAD has increased about 29% in low- and mid-income countries and about 13% in high-income countries Hirsch et al., 2006. It is estimated that approximately 12% of the U.S. adult population is diagnosed with ALI each year. Annually, about 25% of patients will face the risk of death, 30% will face the likelihood of amputation, and 45% will survive with both limbs. At 5 years, more than 60% of patients will die due to ALI Davies, 2012.
ALI is related to a medical condition caused by a sudden lack of blood flow to the hind limbs. This results from a progressive thickening of the arterial lining, caused by buildup of plaque. The plaque, called atherosclerosis, causes stenosis and obstruction of blood flow. Atherosclerosis significantly reduces blood flow to the limbs, such as hands, legs and feet. Critical limb ischemia (CLI) eventually occurs and progresses to the point of rest pain and development of skin ulcers, other ulcers and limb gangrene Norgren et al.,2007. ALI is caused by either embolism or thrombosis. Generally, ALI can be caused by peripheral vascular disease, air, trauma, fat, amniotic fluid, or a tumor Jaffery et al., 2011. Different from stroke or myocardial infarction, ALI usually poses a lower threat of risk to life. Patients must still be treated comprehensively and immediately to re-establish blood flow to the affected areas. To date, almost all therapies for ALI are based on thrombolysis, including the use of streptokinase Andaz et al., 1993Callum and Bradbury, 2000, saline jets, or ultrasonic waves Lyden, 2010. The re-establishment of blood flow may be also done by endovascular interventions, such as artery angioplasty, small balloons or stents, or laser endarterectomy- all which help improve blood flow to the limbs Creager MA, 2008. In the event that endovascular interventions are not effective, a “bypass” around the clot, via insertion of a graft, can be done. However, when reatments fail or when treatment is prolonged or delayed, ALI can result in morbidity, amputation, and/or death Norgren et al.,2007.
In recent years, stem cell therapy has been considered as a promising altenative to treat ALI. Stem cell therapy is promising because it enables the creation of new blood vessels that supply oxygen and nutrients to the ischemic area. Both bone marrow derived mesenchymal stem cells (BM-MSCs) and adipose derived mesenchymal stem cells (AD-MSCs) can be obtained from preclinical Liu et al., 2015Yoshida et al., 2016 and clinical applications Ammar et al., 2015Peeters Weem et al., 2016 to treat ALI. Some studies have used other kinds of stem cells in preclinical trials, including menstrual blood cells Vu et al., 2015, umbilical cord blood derived endothelial progenitor cells Van Pham et al., 2014, placenta derived MSCs Xie et al.,2016Zhang et al., 2014, and Wharton’s jelly derived endothelial progenitor cells Shen et al., 2013.
However, to date, there has not been any study to compare the treatment efficacy of transplanted BM-MSCs versus AD-MSCs for hindlimb ischemia. The aim of this study, therefore, was to compare the efficacy of AD-MSCs and BM-MSCs in allogeneic transplantation for treatment of hindlimb ischemia. The identity of the more suitable source of MSCs is useful not only for hindlimb ischemia treatment but also for angiogenesis therapy.
Materials and methods
Animals
Swiss mice were supplied by the Pasteur Institute in Ho Chi Minh City, Viet Nam. Two to four-week old mice, weighing from 15 to 20 g, were chosen for bone marrow, adipose tissue collection. And 6- to 12-month old mice were used to produce ischemic hindlimb models. All animal protocols and experiments were prepared based on the “Guide for the Care and Use of Laboratory Animals” from the local research institution.
Isolation and culture of AD-MSC
Abdominal fat tissue from mice was collected in phosphate buffered saline (PBS) containing 5% of 100X antibiotic-antimycotic solution (Gibco). All work was performed at biosafety level 2. Adipose tissue was washed twice with PBS and excised into small pieces. Stromal vascular fractions (SVFs) were isolated by an ADSC extraction kit (Geneworld, Viet Nam). Briefly, adipose tissue was washed with Washing Buffer 1 and Washing Buffer 2, respectively. Then, 100 μl of super extract enzyme was added to each mL of fat. The fat was incubated at 380C for 30 min, with shaking for 10 min. Washing Buffer was added to the fat tissue and centrifuged at 3000 RPM for 10 min. After removal of the supernatant, the pellet was rinsed with Washing Buffer three times. SVFs were cultured using an MSCCult kit (Regenmed Lab, Vietnam) and incubated at 370C, with 5% CO2. Fresh medium were replenished every 3 d until the cells reached 70-80% confluency, and cells were passaged several times. The AD-MSCs were harvested, enriched and identified after the 5thpassage.
Isolation and culture of BM-MSC
Mice were used to collect bone marrow derived mononuclear cells (BM-MNCs). MSCs were isolated by culturing BM-MNCs in DMEM/F12 medium (Sigma Aldrich, Louis St, MO) supplemented with 15% fetal bovine serum (FBS, EU Thermo Scientific, Loughborough, UK), 1% of 100X antibiotic-antimycotic solution (Gibco), 10 ng/mL EGF and 10ng/mL FGF (Sigma Aldrich, Louis St, MO). Cells were incubated at 37°C, with 5% CO2. After 3 d, fresh medium was replenished; cell medium was changed every 3 d until the cells reached 70-80% confluency, then the cells were subcultured. The BM-MSCs were identified after the 5th passage.
Characterization of AD-MSCs and BM-MSCs
The AD-MSCs and BM-MSCs were characterized by evaluation of specific expression markers, including cluster of differentiation (CD) 14, CD34, CD44, CD45, CD90, CD105, and CD106. Cells were incubated with antibody at 40°C for 30 min. Flow cytometric data was analyzed with the FACSCalibur machine (BD Biosciences, Franklin Lakes, NJ) and BD CellQuestTM Pro software. Cells were analyzed for their ability to differentiate into adipogenic, osteogenic and chondrogenic lineages. MSCs with a density of 2 x 104 cells/cm2 were plated and incubated at 37°C until the cultures reached a density of about 60-70% confluency.
To confirm the adipogenic potential of the AD-MSCs, cells were cultured in adipogenic induction medium containing DMEM/F12 (Sigma Aldrich, Louis, MO) supplemented with 10% FBS, 1% of 100X antibiotic-antimycotic solution, 10mM dexamethasone, 2.79 mM indomethacine, 5 mg/mL insulin and 0.5 M 1-Methyl-3-isobutylxanthine (IBMX) (Sigma Aldrich, Louis, MO). The differentiation process was visualized by staining with red oil. After 14 d, the cells were fixed with 10% formalin (Sigma Aldrich, Louis, MO) and stained with 0.5% Oil Red O (Sigma Aldrich, Louis, MO).
To confirm the osteogenic potential of AD-MSCs, cells were cultured in osteogenic induction medium containing DMEM/F12 supplemented with 10% FBS (Sigma Aldrich, Louis, MO), 1% of 100X antibiotic-antimycotic solution, 100 nM dexamethasone, 10 mM beta-glycerol, 10 μM ascorbic acid, 20.8 mg/mL L-Leucine and 100 ng/mL L-Lysine. After 31 d, cells were fixed in 10% formalin and stained with 10% Alizarin Red S (Sigma Aldrich, Louis, MO).
Acute hindlimb ischemic mouse model
Acute hindlimb ischemic mice were established according to published protocols of Vu et al., using 6- to 12-month old immunosuppressed mice Vu et al., 2014. Briefly, the mice were anesthetized using 7.5 mg/kg zoletil. Hairy thighs were shaved and an incision, approximately 1 cm long, was made along the thigh skin. The fat thighs were removed and the femoral arteries near the abdomen were dissected from the veins and nerves and ligated at two positions. Between the two ligated artery positions, a burn was made using an electronic cutting machine (ESU-X, Geister, Germany). Finally, the skin was stitched and the wound area was covered in povidone-iodine.
Stem cell transplantation in acute hind limb ischemic mice
Acute hind limb ischemic mice were divided into four groups (15 mice/group). Group 1 contained non-treated mice (without injection). Group 2 contained mice injected with PBS. Group 3 contained mice injected with a dose of 106 AD-MSCs/100 μL PBS, and Group 4 contained mice injected with a dose of 106 BM-MSCs/100 μL PBS. Cells or PBS were directly injected into the muscle at the burn sites. All mice were followed up to 4 weeks to evaluate the effects of the grafted cells.
Evaluation of the recovery of damaged limbs after MSC transplantation
The degree of ischemic damage was assessed through indicators, such as skin color changes, swelling, and grade of the limb necrosis. The grade of limb necrosis was evaluated and classified according to the guidelines, as follows, of Goto et al. Goto et al., 2006: Grade 0, normal limb without swelling, necrosis or atrophy of muscle; Grade I, necrosis limited to the toes; Grade II, necrosis extending to the foot; Grade III, necrosis extending to the knee; and Grade IV, necrosis extending to the hip or loss of whole hind limb. The activity of limbs was evaluated by counting the pedal frequency in water every 10 s and observing the movement of the limb during walking.
Limb edema was assessed at 3 d after cell transplantation. Femoral muscle tissues, weighing 0.1-0.3 g, were collected and the samples were placed in a drying oven at 78°C until a constant weight was observed. The degree of muscle edema was quantified using the wet to-dry weight ratio (W/D), which was compared between untreated and treated mice.
The hindlimb blood flow was evaluated using 0.4% trypan blue. Trypan blue was injected into the tail vein of mouse. The time to foot got blue staning were recorded. The blood flow also was indirectly evaluated based on the saturation of peripheral oxygen (SpO2) by pulse oxymeter was used (Contec, China).
Histological analyses of limb tissues were done at 3d and 28d after cell transplantation. The mice were euthanized and hindlimb muscle was collected. Samples were frozen in optimal cutting temperature compound (Thermo, USA) and cut at cross-sections of 10 μm thickness using a cryostat (Leica, Canada). Tissue section slides were then stained with hematoxylin-eosin (H&E) and assessed by microscopy (Carl-Zeiss, Germany).
The formation of new blood vessels was evaluated during the 4 weeks after cell transplantation. Mice were anesthetized and fixed on a surgical tray and the femoral muscle area was evaluated. The formation of new blood vessels was observed directly with the naked eye and via stereoscopic microscopy. Results were recorded using stereo micrscope (Carl-Zeiss, Germany).
Statistical analysis
All statistical analyses were done using GraphPad Prism 6.0 software. A value of P < 0.05 was considered significant.
Results
Mesenchymal stem cell isolation and characterization
AD-MSCs and BM-MSCs were isolated, grown as primary culture, and then sub-cultured to the 5th passage to obtain homogenous cell populations. In the primary culture, both AD-MSCs and BM-MSCs grew well and reached 70-90% confluence by day 6. Both BM-MSCs ( Figure 1A ) and AD-MSCs ( Figure 1D ) exhibited the fibroblast-like morphology when adhering to the flask surface. Confirmation of harvested MSCs was done by evaluating expression markers as well as assessing potential to differentiate into mesoderm lineages.
In adipogenic-inducing culture medium, oil drops were formed after 7-14 d of induction. The oil droplets grew bigger and were stained red with Oil red solution ( Figure 1B,E ). In osteoblast-inducing culture medium, both BM-MSCs ( Figure 1C ) and AD-MSCs ( Figure 1F ) were able to deposit calcium after 21-30 d of induction; both also stained positive with Alizarin Red staining.
Surface MSC marker expression on both AD-MSCs and BM-MSCs, at the 5th passage, were analyzed by flow cytometry. The data showed that both AD-MSCs and BM-MSCs were positive for CD44, CD90 and CD106, and negative for CD14, CD34, CD45 and CD105 ( Figure 2 ).
Stem cell transplantation and treatment evaluation
Morphology and necrosis grade
The rate of hindlimb recovery was significantly increased in both AD-MSC- and BM-MSC-transplanted mice after 30 d ( Figure 3A,B ). Following treatment, about 76.5% of mice fully recovered (Grade 0) in the AD-MSC group; while only 48.4% of mice recovered in the BM-MSC group (P<0.05). The percentage of mice with grade I/II after treatment was also different between these two groups; the percentage was 23.5% for the AD-MSC group versus 32.3% for the BM-MSC group, respectively. Specifically, there were no mice with grade III/IV in the AD-MSC group, but about 19.4% of mice at this grade in the BM-MSC group.
On the contrary, in the non-treated and PBS groups, there were no mice that fully recovered, and about 10% and 12.5% at grade I, respectively. The majority of mice in the non-treated and PBS groups grew worse, with 90% and 87.5% of the mice at grade III/IV, respectively.
Edema of limbs
At 1d after transplantation, edema appeared in all experimental groups. The process of swelling increased through day 3 and decreased at day 7. During the period from d 3 to d 7, swelling was noted. The majority of untreated mice showed pronounced edema. The edemas were assessed at day 3 by muscle wet-to-dry (W/D) weight ratio ( Figure 3C ). The results showed that edema in both the untreated and PBS groups were significantly higher than the treated groups and in normal mice. At day 3, edema was recorded as: 4.55 ± 0.11 for the untreated group; 4.59 ± 0.08 for PBS group; 3.66 ± 0.68 for AD-MSC group; 3.90 ± 0.58 for BM-MSC group; and 3.37 ± 0.03 for normal mice. At day 3, the edemas of mice in both AD-MSC and BM-MSC transplantation groups were significantly higher than those of normal mice. There was no significant difference between AD-MSC and BM-MSC transplantation groups.
Movement of limbs
All MSC-transplanted mice and non-treated mice moved with difficulty after the first day of transplantation; the mice did not walk well and only dragged their feet. After 30 d, all mice with grade I, II or III damaged-limbs were able to walk fine.
Recovery of blood flow
Recovery of blood flow was assessed by staining the toes of mice with 4% trypan blue dye. For all the experimental groups, grade I damaged-mice were used for evaluation. The results demonstrated that in untreated mice, after 62.44 ± 4.33 seconds of injection of trypan blue dye into the tail vein, both paw pads and toe claws were stained blue ( Figure 4A ). However, the paw pads were not stained with dye in the acute ischemic limb until day 3 ( Figure 4B ); after 28 d, blood circulation was better, and paw pads and toe claws were stained blue after 130.90 ± 7.5 seconds and 122.40 ± 6.77 seconds of injection of trypan blue dye into the tail vein in BM-MSC group and AD-MSC group, respectively ( Figure 4C,D ). These differences were statistically significantly (p<0.05).
Oxyhemoglobin saturation (SpO2)
From the 9th day after transplantation, the recovery of oxygen saturation in AD-MSC and BM-MSC transplanted mouse were above 95%, reached 96.25% ± 1.71% và 96.59%±2.21% (n = 10), respectively. Meanwhile, this value achieved from day 12 in hindlimb ischemic group and PBS group. After 28 days, all experimental group reached normal SPO2 (>98%) ( Figure 5 ).
Structural change of ischemic muscle tissue and formation of new blood vessels
In MSC-transplanted mice, with grade I, II and III damage, after 28 d of treatment there were small blood vessels that appeared around the thigh and the lower limb ( Figure 6 ). Although, the new blood vessels were short compared to control, there were greater numbers. Interestingly, all new blood vessels sprouted from the ends of pre-existing cut vessels.
To evaluate the recovery of damaged skeletal muscle, the skeletal muscles were collected and stained with H&E. The results of H&E staining showed that in the normal mouse, skeletal muscle was arranged in bundles. Cells were arranged in an orderly fashion and the nuclei were located at the periphery of the muscle fiber. Cytoplasmic staining was shown in pink and the cell nuclei staining in purple ( Figure 7 ).
Meanwhile, tissue necrosis occurred in the treated (AD-MSC-transplanted and BM-MSC-transplanted) groups and non-treated group at day 3. The cytoplasm showed shrinkage and tissue structure was rearranged. In some samples, the cells were not arranged with a particular order. The nuclei were not aligned along the edge of the cell (plasma membrane), but were concentrated near or in the cytoplasm. Muscle damage during this period also caused destruction of tissue structures.
However, blood vessel density were higher in AD-MSC and BM-MSC transplanted mouse compare to normal andand non-treated mouse ( Figure 7 and Figure 8 ). On the other hand, the tissue structure of stem cell transplanted-mice were similar to those of normal mice at day 28 of transplantation ( Figure 8 ).
Discussion
Ischemia is a condition related to damage of blood vessels which thereby damage cells by depriving them of oxygen and nutrients. Depending on the location of the broken blood vessels, different organs can be affected resulting in different disease conditions, e.g. hindlimb (ALI), heart (heart attack), and brain (stroke). However, in recent years, therapies have been developed to treat these diseases, though with limited efficacy. Stem cell therapy offers a new strategy for treating these diseases. Unlike previous other therapies, stem cell therapy shows promise for regenerating or creating new blood vessels to bypass the ischemic area. Therefore, such therapies show great potential and are considered the most efficacious for the treatment of ischemia. Both BM-MSCs and AD-MSCs have been evaluated as treatments in both preclinical and clinical applications in previous studies. Although both BM-MSC and AD-MSC transplantation were shown to improve ischemia, there has not been a study to compare the efficacy of transplantation of these kinds of stem cells. Our study shows that AD-MSC transplantation, compared to BM-MSC transplantation, is significantly better for treating ischemic hindlimb in a mouse model.
Firstly, we demonstrated successful isolation of BM-MSCs from bone marrow and AD-MSCs from adipose tissue. Both BM-MSCs and AD-MSCs expressed MSC phenotypic markers. These typical MSC markers included CD44, CD90 and CD105. Both BM-MSCs and AD-MSCs lacked some hematopoietic markers, such as CD34 and CD45. The above markers have been used in previous studies to confirm MSCs from bone marrow Prockop et al., 2001, adipose tissue Caviggioli et al., 2009, and umbilical cord blood Wang et al.,2015. According to Dominici et al., MSCs should exhibit some of these properties: a fibroblast-like shape; expression of CD44, CD73, and CD90, while negative for CD14, CD34, and CD45; and successful differentiation into some mesoderm cells, such as adipocytes, osteoblasts and chondroblasts Dominici et al.,2006.
We demonstrated that both BM-MSCs and AD-MSCs successfully differentiated into osteoblasts and adipocytes. After 14-21 d of differentiation, the stored oil droplets in the cytoplasm and these oil droplets could be stained with oil red dye. Moreover, after 14-21 d of differentiation, the cells accumulated calcium and stained positive with alizarin red staining. Taken together, the data show that both BM-MSCs and AD-MSCs exhibited the characteristic MSC phenotypes.
These stem cells were then assessed for their ability to treat hind limb ischemia. Using the protocol of Niiyama et al. Niiyama et al., 2009, we successfully established grade IV hind limb ischemia in mice. After 48–72 h, necrosis extended to the knee, and trypan blue infusion showed that there was no blood supply to any blood vessel in the hind limb. After 96–120 h of artery ligation, all mice lost their legs.
The treatment results showed that both AD-MSC and BM-MSC transplantation significantly improved the treatment efficacy of hindlimb ischemia, compared to controls (non-treated and PBS groups). Mice in both AD-MSC and BM-MSC groups significantly improved necrosis of the hindlimb, had decreased edema, formed some new blood vessels, and showed restructuring of skeletal muscles at the injured sites. Interestingly, there was 0% of mice in the un-treated and PBS groups that fully recovered but 76.5%; and 48.4% of fully recovered mice in the AD-MSC and BM-MSC groups, respectively. Along with the other observations, including edema, histological evaluation, and new blood vessel formation, the data showed that AD-MSC transplantation was superior over BM-MSC transplantation.
The data also indicate that BM-MSCs and AD-MSCs have an important role in the regeneration. Neovascularization and angiogenesis are the two main processes in recovery of ischemia.
Vasculogenesis is the process that forms vascular structures from circulating or tissue-resident endothelial stem cells (angioblasts) which proliferate into de novo endothelial cells. Angiogenesis relates to the formation of thin-walled endothelial-lined structures with a vascular smooth muscle wall and of pericytes. From our observation of blood vessels after 30 d of stem cell transplantation, it can be deduced that the angiogenesis (new vessel formation from pre-existing vessels) was the predominant process.
MSCs can contribute to angiogenesis via secreted factors. In fact, in allogeneic transplantation of MSCs, almost all MSCs will be removed from the body by the host’s immune system. In recent studies, Shen et al. showed that BM-MSCs could produce VEGF Shen et al., 2016. BM-MSCs also secrete IL-8 which promotes angiogenesis Wang et al., 2015. AD-MSCs produce and secrete many angiogenic factors, such as VEGF Lauvrud et al., 2016 and HGF Suga et al., 2009.
Moreover, our results clearly showed that AD-MSCs was superior over BM-MSCs at significantly improving hindlimb ischemia; the percentage of fully recovered mice (grade 0) after 30 d of treatment was 76.5% in AD-MSC group compared to 48.4% in the BM-MSC group. This difference could relate to some paracrine factors which BM-MSCs and AD-MSCs may produce during interactions of BM-MSCs and AD-MSCs with endothelial cells or injured cells at the hindlimb. In vitro, BM-MSC and AD-MSCs exhibited different responses to endothelial cells; this has been shown in previous studies. Verseijden et al. has shown that BM-MSCs can produce HGF, TIMP1, and TIMP2, promoting angiogenesis Verseijden et al., 2010. AD-MSCs can produce growth factors and cytokines such as HGF, TNF-alpha Kachgal and Putnam, 2011, PD-ECGF, FGF-2, MMP-9, Ang-2, pentraxin-3 Rohringer et al., 2014, collagen IV, and fibronectin Merfeld-Clauss et al., 2010. AD-MSCs have also been shown to have a high proliferative potential than BM-MSCs Li et al., 2015.
Lotfy et al. showed that while there are similarities between BM-MSCs and AD-MSCs, their main difference lies in their application for specific diseases Lotfy et al., 2014. AD-MSCs seem more promising for regenerative application, in general. However, BM-MSCs may represent a better choice for treating bone disorders Lotfy et al., 2014. Liao et al. have reviewed and compared the bone formation between AD-MSCs and BM-MSCs and showed that there are no significant differences between these two kinds of stem cells Liao and Chen, 2014. However, under in vitro and in vivo conditions, AD-MSCs supported hematopoiesis better than BM-MSCs Nakao et al., 2010. Hao et al. compared the effects of freshly isolated SVF and BM-MNC for transplantation in mouse hindlimb models. The result showed similar effects between SVFs and MNCs in hindlimb ischemia. However, the main difference of our study with Hao’s study is that we used cultured MSCs, while Hao et al. used non-expanded cells (SVFs and MNCs).
Although, there has not been a comparative study of BM-MSCs and AD-MSCs in hindlimb ischemia treatment, some comparative studies in other diseases have been done. In a current study, BM-MSCs and AD-MSCs were used to treat DOX-induced cardiac damage; Ammar et al. showed that both BM-MSCs and AD-MSCs could be used to treat the disease with equal efficacy. Both MSCs affected cardiac damage by promoting angiogenesis, decreasing infiltration of immune cells and impacting collagen deposition (Ammar et al., 2015). In another study, Hiwatashi et al. compared the effects of BM-MSCs and AD-MSCs in combination with atelocollagen for vocal fold scar regeneration. The authors showed that AD-MSCs had more potential in terms of restoration of hyaluronic acid and suppression of excessive collagen deposition Hiwatashi et al.,2014Hiwatashi et al., 2016. Both BM-MSCs and AD-MSCs were considered to have similar potential for treating methotrexate-induced pulmonary fibrosis in rat Fikry et al., 2015. In a rabbit model of tendon repair, AD-MSCs also exhibited greater treatment potential than BM-MSCs Behfar et al., 2014.
A limitation in our study was the lack of Dopler ultrasound to evaluate and monitor blood stream into the hindlimb during the regeneration process. However, the evidence from the necrosis grade, edema level, muscle restructure and blood formation provided initial demonstration that AD-MSC transplantation is superior over BM-MSC transplantation. Our study shows that AD-MSC transplantation may be significantly better for treatment of hindlimb ischemia.
Conclusion
Stem cell therapy is considered a promising therapy for some ischemic diseases, including hindlimb ischemia. Both BM-MSCs and AD-MSCs are promising sources of MSCs for treating ALI. Our study showed that AD-MSCs has greater therapeutic potential than BM-MSCs to treat hindlimb ischemia. AD-MSC implantation led to strong fully recovery of hindlimb ischemia in mice. Although BM-MSCs also exhibited strong angiogenic potential in the hindlimb ischemia mouse model, the percentage of fully recovered mice was significantly lower than that for AD-MSC implantation. Moreover, the procedure for collecting BM is more clinically invasive than adipose tissue collection. Taken together, our results suggest that AD-MSCs are the more suitable source of MSCs for ischemia treatment as well as angiogenesis therapy.
References
-
H.I.
Ammar,
G.L.
Sequiera,
M.B.
Nashed,
R.I.
Ammar,
H.M.
Gabr,
H.E.
Elsayed,
N.
Sareen,
E.A.
Rub,
M.B.
Zickri,
S.
Dhingra.
Comparison of adipose tissue- and bone marrow- derived mesenchymal stem cells for alleviating doxorubicin-induced cardiac dysfunction in diabetic rats. Stem Cell Res Ther.
2015;
6
:
14–8
.
-
S.
Andaz,
D.A.
Shields,
J.H.
Scurr,
P.D.
Smith.
Thrombolysis in acute lower limb ischaemia. Eur J Vasc Surg.
1993;
7
:
595-603
.
-
M.
Behfar,
S.
Javanmardi,
F.
Sarrafzadeh-Rezaei.
Comparative study on functional effects of allotransplantation of bone marrow stromal cells and adipose derived stromal vascular fraction on tendon repair: a biomechanical study in rabbits. Cell J.
2014;
16
:
263-270
.
-
K.
Callum,
A.
Bradbury.
ABC of arterial and venous disease: Acute limb ischaemia. BMJ.
2000;
320
:
764-767
.
-
F.
Caviggioli,
V.
Vinci,
A.
Salval,
M.
Klinger.
Human adipose-derived stem cells: isolation, characterization and applications in surgery. ANZ J Surg.
2009;
79
:
856
.
-
L.J.
Creager MA.
Vascular diseases of the extremities. In: Fauci AS, Braunwald E, Kasper DL, et al., editors. Harrison’s Principles of Internal Medicine.. 17th ed. New York, NY, USA: McGraw Hill.
2008
.
-
M.G.
Davies.
Criticial Limb Ischemia: Epidemiology. Methodist DeBakey Cardiovascular Journal.
2012;
8
:
10-14
.
-
M.
Dominici,
K.
Le Blanc,
I.
Mueller,
I.
Slaper-Cortenbach,
F.
Marini,
D.
Krause,
R.
Deans,
A.
Keating,
D.
Prockop,
E.
Horwitz.
Minimal criteria for defining multipotent mesenchymal stromal cells. The International Society for Cellular Therapy position statement.. Cytotherapy.
2006;
8
:
315-317
.
-
E.M.
Fikry,
M.M.
Safar,
W.A.
Hasan,
H.M.
Fawzy,
E.E.
El-Denshary.
Bone Marrow and Adipose-Derived Mesenchymal Stem Cells Alleviate Methotrexate-Induced Pulmonary Fibrosis in Rat: Comparison with Dexamethasone. J Biochem Mol Toxicol.
2015;
29
:
321-329
.
-
T.
Goto,
N.
Fukuyama,
A.
Aki,
K.
Kanabuchi,
K.
Kimura,
H.
Taira,
E.
Tanaka,
N.
Wakana,
H.
Mori,
H.
Inoue.
Search for appropriate experimental methods to create stable hind-limb ischemia in mouse. The Tokai journal of experimental and clinical medicine.
2006;
31
:
128-132
.
-
A.T.
Hirsch,
Z.J.
Haskal,
N.R.
Hertzer,
C.W.
Bakal,
M.A.
Creager,
J.L.
Halperin,
L.F.
Hiratzka,
W.R.
Murphy,
J.W.
Olin,
J.B.
Puschett.
ACC/AHA 2005 Practice Guidelines for the management of patients with peripheral arterial disease (lower extremity, renal, mesenteric, and abdominal aortic): a collaborative report from the American Association for Vascular Surgery/Society for Vascular Surgery, Society for Cardiovascular Angiography and Interventions, Society for Vascular Medicine and Biology, Society of Interventional Radiology, and the ACC/AHA Task Force on Practice Guidelines (Writing Committee to Develop Guidelines for the Management of Patients With Peripheral Arterial Disease): endorsed by the American Association of Cardiovascular and Pulmonary Rehabilitation; National Heart, Lung, and Blood Institute; Society for Vascular Nursing; TransAtlantic Inter-Society Consensus; and Vascular Disease Foundation. Circulation.
2006;
113
:
e463-654
.
-
N.
Hiwatashi,
S.
Hirano,
M.
Mizuta,
I.
Tateya,
S.
Kanemaru,
T.
Nakamura,
J.
Ito.
Adipose-derived stem cells versus bone marrow-derived stem cells for vocal fold regeneration. Laryngoscope.
2014;
124
:
E461-469
.
-
N.
Hiwatashi,
S.
Hirano,
R.
Suzuki,
Y.
Kawai,
M.
Mizuta,
Y.
Kishimoto,
I.
Tateya,
S.
Kanemaru,
T.
Nakamura,
M.
Dezawa.
Comparison of ASCs and BMSCs combined with atelocollagen for vocal fold scar regeneration. Laryngoscope.
2016;
126
:
1143-1150
.
-
Z.
Jaffery,
S.N.
Thornton,
C.J.
White.
Acute limb ischemia. Am J Med Sci.
2011;
342
:
226-234
.
-
S.
Kachgal,
A.J.
Putnam.
Mesenchymal stem cells from adipose and bone marrow promote angiogenesis via distinct cytokine and protease expression mechanisms. Angiogenesis.
2011;
14
:
47-59
.
-
A.T.
Lauvrud,
P.
Kelk,
M.
Wiberg,
P.J.
Kingham.
Characterization of human adipose tissue-derived stem cells with enhanced angiogenic and adipogenic properties. J Tissue Eng Regen Med.
2016
.
-
C.Y.
Li,
X.Y.
Wu,
J.B.
Tong,
X.X.
Yang,
J.L.
Zhao,
Q.F.
Zheng,
G.B.
Zhao,
Z.J.
Ma.
Comparative analysis of human mesenchymal stem cells from bone marrow and adipose tissue under xeno-free conditions for cell therapy. Stem Cell Res Ther.
2015;
6
:
55
.
-
H.T.
Liao,
C.T.
Chen.
Osteogenic potential: Comparison between bone marrow and adipose-derived mesenchymal stem cells. World J Stem Cells.
2014;
6
:
288-295
.
-
J.
Liu,
H.
Hao,
L.
Xia,
D.
Ti,
H.
Huang,
L.
Dong,
C.
Tong,
Q.
Hou,
Y.
Zhao,
H.
Liu.
Hypoxia pretreatment of bone marrow mesenchymal stem cells facilitates angiogenesis by improving the function of endothelial cells in diabetic rats with lower ischemia. PLoS One.
2015;
10
:
e0126715
.
-
A.
Lotfy,
M.
Salama,
F.
Zahran,
E.
Jones,
A.
Badawy,
M.
Sobh.
Characterization of mesenchymal stem cells derived from rat bone marrow and adipose tissue: a comparative study. Int J Stem Cells.
2014;
7
:
135-142
.
-
S.P.
Lyden.
Endovascular treatment of acute limb ischemia: review of current plasminogen activators and mechanical thrombectomy devices. Perspect Vasc Surg Endovasc Ther.
2010;
22
:
219-222
.
-
S.
Merfeld-Clauss,
N.
Gollahalli,
K.L.
March,
D.O.
Traktuev.
Adipose tissue progenitor cells directly interact with endothelial cells to induce vascular network formation. Tissue Eng Part A.
2010;
16
:
2953-2966
.
-
D.
Mozaffarian,
E.J.
Benjamin,
A.S.
Go,
D.K.
Arnett,
M.J.
Blaha,
M.
Cushman,
S.R.
Das,
S.
de Ferranti,
J.P.
Despres,
H.J.
Fullerton.
Heart Disease and Stroke Statistics-2016 Update: A Report From the American Heart Association. Circulation.
2016;
133
:
e38–e360
.
-
N.
Nakao,
T.
Nakayama,
T.
Yahata,
Y.
Muguruma,
S.
Saito,
Y.
Miyata,
K.
Yamamoto,
T.
Naoe.
Adipose tissue-derived mesenchymal stem cells facilitate hematopoiesis in vitro and in vivo: advantages over bone marrow-derived mesenchymal stem cells. Am J Pathol.
2010;
177
:
547-554
.
-
H.
Niiyama,
N.F.
Huang,
M.D.
Rollins,
J.P.
Cooke.
Murine model of hindlimb ischemia. J Vis Exp.
2009
.
-
L.
Norgren,
W.R.
Hiatt,
J.A.
Dormandy,
M.R.
Nehler,
K.A.
Harris,
F.G.
Fowkes.
Inter-Society Consensus for the Management of Peripheral Arterial Disease (TASC II). Journal of vascular surgery 45 Suppl S.
2007;
:
S5-67
.
-
S.M.
Peeters Weem,
M.
Teraa,
H.M.
den Ruijter,
G.J.
de Borst,
M.C.
Verhaar,
F.L.
Moll.
Quality of Life After Treatment with Autologous Bone Marrow Derived Cells in No Option Severe Limb Ischemia. Eur J Vasc Endovasc Surg.
2016;
51
:
83-89
.
-
D.J.
Prockop,
I.
Sekiya,
D.C.
Colter.
Isolation and characterization of rapidly self-renewing stem cells from cultures of human marrow stromal cells. Cytotherapy.
2001;
3
:
393-396
.
-
S.
Rohringer,
P.
Hofbauer,
K.H.
Schneider,
A.M.
Husa,
G.
Feichtinger,
A.
Peterbauer-Scherb,
H.
Redl,
W.
Holnthoner.
Mechanisms of vasculogenesis in 3D fibrin matrices mediated by the interaction of adipose-derived stem cells and endothelial cells. Angiogenesis.
2014;
17
:
921-933
.
-
C.C.
Shen,
B.
Chen,
J.T.
Gu,
J.L.
Ning,
L.
Chen,
J.
Zeng,
B.
Yi,
K.Z.
Lu.
The angiogenic related functions of bone marrow mesenchymal stem cells are promoted by CBDL rat serum via the Akt/Nrf2 pathway. Exp Cell Res.
2016
.
-
W.C.
Shen,
C.J.
Liang,
V.C.
Wu,
S.H.
Wang,
G.H.
Young,
I.R.
Lai,
C.L.
Chien,
S.M.
Wang,
K.D.
Wu,
Y.L.
Chen.
Endothelial progenitor cells derived from Wharton’s jelly of the umbilical cord reduces ischemia-induced hind limb injury in diabetic mice by inducing HIF-1alpha/IL-8 expression. Stem Cells Dev.
2013;
22
:
1408-1418
.
-
H.
Suga,
H.
Eto,
T.
Shigeura,
K.
Inoue,
N.
Aoi,
H.
Kato,
S.
Nishimura,
I.
Manabe,
K.
Gonda,
K.
Yoshimura.
IFATS collection: Fibroblast growth factor-2-induced hepatocyte growth factor secretion by adipose-derived stromal cells inhibits postinjury fibrogenesis through a c-Jun N-terminal kinase-dependent mechanism. Stem Cells.
2009;
27
:
238-249
.
-
P.
Van Pham,
N.-T.A.
Bui,
N.-L.
Trinh,
T.L.
Phi,
K.N.
Phan,
B.N.
Vu.
A comparison of umbilical cord blood-derived endothelial progenitor and mononuclear cell transplantation for the treatment of acute hindlimb ischemia. Biomedical Research and Therapy.
2014;
1
:
1-12
.
-
F.
Verseijden,
S.J.
Posthumus-van Sluijs,
P.
Pavljasevic,
S.O.
Hofer,
G.J.
van Osch,
E.
Farrell.
Adult human bone marrow- and adipose tissue-derived stromal cells support the formation of prevascular-like structures from endothelial cells in vitro. Tissue Eng Part A.
2010;
16
:
101-114
.
-
N.B.
Vu,
A.N.-T.
Bui,
V.
Ngoc-Le Trinh,
L.T.
Phi,
N.K.
Phan,
P.
Van Pham.
A comparison of umbilical cord blood-derived endothelial progeni-tor and mononuclear cell transplantation for the treatment of acute hindlimb ischemia. 2014
.
-
N.B.
Vu,
V.
Ngoc-Le Trinh,
L.T.
Phi,
N.K.
Phan,
P.
Van Pham.
Human menstrual blood-derived stem cell transplantation for acute hind limb ischemia treatment in mouse models.. In Regenerative Medicine (Springer).
2015;
:
205-215
.
-
J.
Wang,
Y.
Wang,
S.
Wang,
J.
Cai,
J.
Shi,
X.
Sui,
Y.
Cao,
W.
Huang,
X.
Chen,
Z.
Cai.
Bone marrow-derived mesenchymal stem cell-secreted IL-8 promotes the angiogenesis and growth of colorectal cancer. Oncotarget.
2015;
6
:
42825-42837
.
-
N.
Xie,
Z.
Li,
T.M.
Adesanya,
W.
Guo,
Y.
Liu,
M.
Fu,
A.
Kilic,
T.
Tan,
H.
Zhu,
X.
Xie.
Transplantation of placenta-derived mesenchymal stem cells enhances angiogenesis after ischemic limb injury in mice. J Cell Mol Med.
2016;
20
:
29-37
.
-
S.
Yoshida,
H.
Yoshimoto,
A.
Hirano,
S.
Akita.
Wound Healing and Angiogenesis through Combined Use of a Vascularized Tissue Flap and Adipose-Derived Stem Cells in a Rat Hindlimb Irradiated Ischemia Model. Plast Reconstr Surg.
2016;
137
:
1486-1497
.
-
B.
Zhang,
T.M.
Adesanya,
L.
Zhang,
N.
Xie,
Z.
Chen,
M.
Fu,
J.
Zhang,
J.
Zhang,
T.
Tan,
A.
Kilic.
Delivery of placenta-derived mesenchymal stem cells ameliorates ischemia induced limb injury by immunomodulation. Cell Physiol Biochem.
2014;
34
:
1998-2006
.
Comments
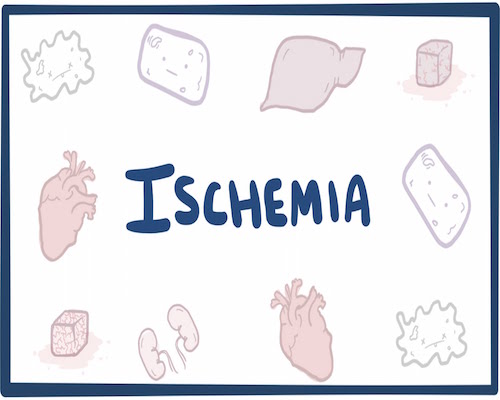
Downloads
Article Details
Volume & Issue : Vol 3 No 09 (2016)
Page No.: 844-856
Published on: 2016-09-29
Citations
Copyrights & License

This work is licensed under a Creative Commons Attribution 4.0 International License.
Search Panel
Pubmed
Google Scholar
Pubmed
Google Scholar
Pubmed
Google Scholar
Pubmed
Google Scholar
Pubmed
Google Scholar
Pubmed
Search for this article in:
Google Scholar
Researchgate
- HTML viewed - 7777 times
- Download PDF downloaded - 1936 times
- View Article downloaded - 13 times