A mouse model of osteonecrotic femoral head induced by methylprednisolone and lipopolysaccharide
Abstract
Introduction: Osteonecrosis of the femoral head is caused by various factors, including prolonged use of steroid drugs, use of alcohol, vascular injuries and hemoglobinopathies. This study aims to develop a mouse model for glucocorticoid-induced avascular necrosis (AVN) of the femoral head.
Methods: Adult mice were randomly divided into two groups: experimental and control. Group A (the experimental group) was given (via intramuscular injection) 10 mg/kg of lipopolysaccharide (LPS) and 30 mg/kg of methylprednisolone (MPS). Each mouse additionally received MPS in divided oral doses of 13 mg/kg for 10 consecutive days. Group B (the control group) received normal saline at the same location and same volume as those in Group A. Histological changes of the femoral heads were observed by electron microscopy at 3, 5, and 7 weeks after the last chemical injection. The percentage of empty lacunae was measured randomly and the expression of fibrocartilage was evaluated using an image analyzing system. The expression of CD31 and VEGF-R2 were observed by immunohistochemistry. The bone marrow-derived mononuclear cells were stained with propidium iodide and cell cycle was analyzed by flow cytometry.
Results: The results showed that at weeks 3 and 5, mice in Group A showed an increase in body weight. From weeks 5 to 7, mouse body weight in both groups remained constant. No difference in bone morphology was observed at week 7. The percentage of empty lacunae was 5.87 2.49% at week 5 and 21.58 8.10% at week 7. After 7 weeks, chondrocyte degeneration and fibrocartilage expression were observed. Moreover, the density of CD31 and VEGF-R2 markers increased in the femoral head. The rate of apoptosis in the bone marrow increased at week 3 then decreased.
Conclusion: The data show that MPS, combined with LPS, can induce in mice features typical of early AVN of the femoral head.
Introduction
AVN (also called osteonecrosis) of the femoral head is defined as a pathological state with death and/or necrosis of bone marrow cells and osteocytes due to interruption of blood supply to the bone Kerachian et al., 2010a. This leads to the collapse of the necrotic segment. Factors that may cause AVN include steroid administration, alcohol abuse, hemoglobinopathies, traumatic events, vascular injury and idiopathic origins J. Anthony Parker, 1988. In the early stage, patients do not show distinct symptoms but structural and cellular changes can be observed at the histological level. To date, treatment for this disease includes non-weight bearing therapy, bone grafting (for physical support) and tantalum implants Zalavras et al., 2003b.
Glucocorticoids are absorbed through the cell membrane by special receptors and affect lipid, protein and carbohydrate metabolism. The effect of glucocorticoids on bone can be summarized by three major mechanisms: (i) the imbalance between bone resorption and bone remodeling, (ii) vascular occlusion or vascular injury, and (iii) activation of apoptotic signaling pathway by some glucocorticoids Zalavras et al.,2003b. At the cellular level, glucocorticoids can decrease mitosis, increase apoptosis by Fas pathway and inhibit type II collagen synthetic ability in osteoblasts. For osteoclasts, glucocorticoids increase the expression of differentiation cytokines, e.g. G-CSF, RANK-L and IL-6. Similar to their effect on osteoblasts, glucocorticoids can stimulate apoptosis of osteocytes and change their elastic modulus. In addition, high levels of glucocorticoids can cause inhibition of lymphoid enhancer-binding factor 1/transcription factor 7 (LEF/TCF), associated with beta-catenin, in canonical Wnt signaling pathway MacDonald et al., 2009; therefore, the transition from G1 to S phase can be restricted (Ichiseki et al.). Moreover, glucocorticoids can cause reduced growth of cartilage and reduced synthesis of collagen and proteoglycans. Many animal models of AVN have been established using different glucocorticoids, such as prednisolone Qian et al.,2008, methylprednisolone (MPS) Ichiseki et al., 2004b, and dexamethasone Yin et al., 2006. Among them, MPS, a synthetic glucocorticoid, has been demonstrated to effectively increase osteonecrosis Miyanishi et al., 2005.
Lipopolysaccharides (LPS), molecules found in the outer membrane of gram-negative bacteria, have immunostimulatory properties; they can induce systemic inflammation and sepsis through Toll-like Receptor 4 Beutler and Rietschel, 2003. Therefore, application of LPS in our model should affect cartilage Bobacz et al., 2007Ichiseki et al., 2004a. Several studies have demonstrated that LPS activates many transcription factors and cytokines, including NF-κB, IL-33 Espinassous et al., 2009. In fact, the bone marrow and immune system are functionally interconnected Zupan et al., 2013. Thus, cellular immune and pro-inflammatory cytokines can impact bone cells. Recent studies have reported that LPS can enhance a glucocorticoidinduced disease progression model Qin et al., 2006Ryoo et al., 2014Wu et al., 2008.
In this study, AVN of the femoral head was induced by combining LPS and MPS in an effort to develop an experimental mouse model. Development of such a model will be useful for evaluation of effective therapeutic modalities for AVN.
Materials and methods
Establishment of a Mouse Model of AVN
Adult mice (ranging from 4-8 weeks in age and 25 – 30 grams in weight) were randomly divided into two groups. Group A (experimental group) received intravenous injection of 10 mg/kg LPS and 30 mg/kg MPS ( Table 1 ). Moreover, each mouse received an additional administration of Medrol (Pfizer, Italy), given orally in divided doses of 13 mg/kg for 10 days. Group B (control group) received normal saline; the injection location and volume were the same as those used for Group A. The animals were evaluated at weeks 3, 5 and 7, after the last injection.
Histological Assay
Femurs were collected from sacrificed mice and fixed in paraformaldehyde (4%) for 2-3 days. To demineralize the calcium, femurs were transferred to phosphate buffered saline containing 10% disodium ethylene diamine triacetic acid (PBS-EDTA) for 10-14 days or until the femurs were tender. The femurs were then dehydrated by glucose (10%) and later embedded in tissue freezing medium and cut into 10-15 μm-thick sections. Finally, samples were stained with hematoxylin and eosin dye and observed by microscopy. For each section, several random fields were observed and evaluated for number of bone lacunae. The proportion of empty lacunae to bone lacunae was calculated.
To evaluate the expression of proteoglycans, the samples were stained with Safranin O. Images were analyzed by imageJ software (National Institutes of Health, USA).
Immunohistochemistry
For detection of VEGF and CD31 expression (in the femoral head following drug treatment) immunohistochemistry was used. Tissues were sectioned according to the histological assay mentioned above, and stained with Rat-anti mouse VEGF-R2 (Flk1) FITC (BD Bioscience, US) and Rat-anti mouse CD31 FITC (BD Bioscience, US). Hoechst dye was used for nuclear staining. The results were observed by fluorescent microscopy.
Cell cycle assay
Bone marrow-derived mononuclear cells were collected by incubation in hemolysis solution. Mononuclear cells were fixed in ethanol (70%) for 24 hours and stained with propidium iodide for 10 minutes. Cell cycle analysis was done by flow cytometry.
Statistical Analysis
The data were analyzed by GraphPad Prism 6.0 software and Microsoft Office 2011. Images were analyzed by ImageJ software.
Results
Weight change
Weight of mice in both groups increased over time. However, the rate of weight gain was faster in the experimental group. The rate of weight gain was approximately 19.63% ± 8.29% at week 3 and 30.01% ± 13.55% at week 5, compared to the control group (14.42% ± 5.69%). From weeks 5 to 7, the body weight of mice in both groups remained constant.
Morphological changes of the cartilage and bone
To observe the occurrence of AVN of the femoral head, we used microscopy and several cytological and histological examinations. After 7 weeks of drug administration, bone morphology showed no significant change in treated mice compared to control mice, by observation with the naked eye and by stereoscopic microscopy ( Figure 1A ). However, the femoral head of drug-treated mice changed to an opaque color, whereas the femoral head in control mice remained white in color ( Figure 1B ). Moreover, there were dents present on the femoral head of drug-induced mice ( Figure 1C ).
Bone lacunae
The loss of osteocytes in bone tissue is one evident change which indicates AVN of the femoral head Ichiseki et al., 2004a. Our study showed that the percentage of empty lacunae gradually increased from week 3 to week 7. While the empty bone lacunae in the control group at week 3 was 0.28% ± 0.28% ( Figure 2A ), the ratio in the drug-treated group was 0.68% ± 0.16%, and reached 5.87% ± 2.49% in week 5 and 21.58% ± 8.10% in week 7 ( Figure 2B ) (p<0.05). The rate of bone defect at week 3 was not different compared to control mice ((p>0.05), but there was a statistically significant difference in the groups at week 5 and also at week 7 (p<0.05).
Histological Assay
The histological features of chondrocytes at the femur head looked distinctly different in the control group versus drug-treated group, at week 7. In the control tissue, the normal cartilage cells were inlaid in the chamber with tight contact structures (blue arrow) ( Figure 3A ). In the drug-treated tissue, there were visible hollow cavities between the chondrocytes, indicative of loss of tissue structure, and the cartilage cells looked injured and atrophic (blue arrow) ( Figure 3B ).
The distribution of proteoglycan
Cartilage samples were stained by safranin O dye ( Figure 4A , Figure 4B ). The proteoglycan content in normal cartilage was stained orange to red, fibrocartilage was stained green, and nuclei were stained black. Proteoglycan expression was recorded by analyzing the digital photomicrographs via ImageJ software. Statistical results showed the proteoglycan expression level in the normal (control) group to be at 2.63 ± 0.47 while only at 0.98 ± 0.18 in the drug-induced group, after 7 weeks. This indicated a statistically significant reduction in the amount of proteoglycan expression in mice, after treatment with LPS and MPS (p<0.001) ( Figure 4C ).
Cell cycle assay
There was a slight decrease in the G0/G1-phase of the cell cycle in the treatment group at week 3 (84.11% ± 4.86%), compared to the control group (85.36% ± 2.78%). These changes continued post week 3 (81.23% ± 6.47%) through week 7 (81.89% ± 5.30%). However, statistical analysis showed no significant difference observed in the G0-G1 phase of cell cycle arrest during the drug induction process ( Figure 5A , Figure 5D ).
The main changes were found in the proliferative phase (S-G2/M phase) and apoptotic phase. The percentage of cell cycle arrest at G0/G1 phase increased significantly (p<0.05) in the 5-week and 7-week drug-induced group (17.69% ± 3.59% and 17.62% ± 4.11%, respectively), compared to the 3-week drug-induced group (11.78% ± 2.88%) and control group (13.66% ± 3.56%) ( Figure 5B ).
The mononuclear cells in drug-induced bone marrow showed an increased level of apoptosis (p<0.05) at week 3 (4.11% ± 4.60%), compared to cells in control mice at the same time point (0.97% ± 0.53%). After 3 weeks, the level of apoptosis gradually reduced ( Figure 5C , Figure 5E ).
Immunohistochemistry
Expression of vascular endothelial growth factor receptor 2 (VEGF-R2) and CD31 in chondrocytes were also evaluated by immunohistochemistry; nuclei were indicated by Hoechst staining. VEGF-R2 and CD31 protein levels were assessed to determine damage to cartilage and vascular structures. Immunohistochemistry analysis showed that VEGFR-2 and CD31 signals were scattered in cartilage tissue. The expression of CD31 in the normal mouse (control group) was lower than in the drug-induced group at week 7 ( Figure 6 ). VEGFR-2 protein expression is different in control mice compared to drug-induced mice ( Figure 7 ).
Discussion
A decrease in chondrocyte numbers may be the result of injury, induced by mechanical damage or steroid drug use Chen et al., 2001. Cartilage injury leads to apoptosis or necrosis and can contribute to changes in tissue structure. MPS and LPS have been demonstrated to induce change in cartilage structure. Many glycosaminoglycans attach to proteoglycans Esko JD et al., 2009, which are secreted into the cartilage matrix by chondrocytes. The interaction between chondrocytes and cartilage matrix play an important role in proliferation and cartilage remodeling.
MPS causes a reduction in size of aggrecans or aggregate proteoglycan in the cartilage matrix. This leads to the loss of glycosaminoglycans Todhunter et al., 1996. LPS activates TLR-4, a receptor for LPS which has been identified in chondrocytes. Upon LPS-induced TLR-4 activation, enhanced phosphorylation of p38 MAPK can occur; some studies have shown that activation of p38 MAPK leads to decrease of GAG synthesis in chondrocytes in vitro. In addition, enhanced regulation of IL-1β, controlled by p38 MAPK, inhibits the production of cartilage matrix Azuma et al., 2000and consequently to the loss of matrix structure.
Moreover, Nakazawa et al. showed that apoptosis increases in corticosteroid-induced chondrocytes Nakazawa et al., 2002. The membrane of chondrocytes partly expresses Fas antigen therefore stimulation of Fas receptor can induce apoptosis of chondrocytes Lotz et al., 1999.As a result, chondrocytes disappear and fibrochondrocytes develop in drug-treated cartilage.
Collagen is distributed in almost all connective tissue. Type I collagen is the major protein in bone and occupies approximately 80% of the total proteins present in bone Wu and Eyre, 1995. Collagen has mechanical functions and plays a substantial role in its toughness. Bone collagen abnormalities can lead to bone erosion Viguet-Carrin et al., 2006. LPS and MPS have been reported to inhibit collagen and non-collagen protein synthesis in bone matrix Larjava et al., 1987Millar et al., 1986. Moreover, researchers have shown that MPS inhibits synthesis of Wnt; since Wnt-mediated signals are involved in bone formation and resorption Kobayashi et al., 2008, the inhibition of Wnt can lead to decreased bone formation [24]. Bone erosion may be also caused by negative regulation of Fas expression, which extends the life span of osteoclasts Wu et al., 2003.
LPS and MPS impact the immune system as well as proliferation of bone cells. The bone marrow cell populations include many different types, such as stem cells, adipocytes, progenitor endothelial cells and immune cells. LPS and MPS can cause apoptosis in bone marrow-derived cells, particularly immune cells. LPS induces macrophage apoptosis through two mechanisms: secretion of TNF-α (early apoptosis) and production of nitric oxide (late apoptosis) Azuma et al., 2000. Through the Fas signaling pathway, MPS promotes apoptosis of bone cells Zalavras et al., 2003a. Glucocorticoids also rapidly induce apoptosis of T cells, B cells, neutrophils and eosinophils McManus, 2003Walsh, 2000. In this study, apoptosis was clearly induced in these cells after MPS and LPS administration.
Corticosteroids have been reported to stimulate osteoclast formation and lacunar resorption Hirayama et al.,2002. LPS and MPS stimulate immune cells to produce many different types of cytokines which affect osteoclasts Stamatos et al., 2010. There are three cytokine groups: (i) cytokines which activate osteoclasts Shaarawy et al., 2003, (ii) cytokines which inhibit osteoclast activity van't Hof and Ralston, 1997, and (iii) cytokines which have dual action on osteoclasts Zupan et al., 2013. Some cytokines, such as IL1, TNF-α, prostaglandin E2, G-CSF and RANK-L, induce the differentiation and activity of osteoclasts Azuma et al.,2000Sato and Takayanagi, 2006. In contrast, cytokines like interleukin-4, gamma-interferon and transforming factor-beta inhibit both osteoclast formation and osteoclast activity Roodman, 1993. LPS binds to osteoblasts in bone through CD14 receptor and stimulates them to produce cytokines and eicosanoids, thereby activating osteoclasts Itoh et al., 2003. Osteoclasts are formed by the fusion of many cells derived from blood, such as mature monocytes and macrophagesUdagawa et al., 1990.
In this study, LPS and MPS stimulated the division of bone marrow-derived mononuclear cells, beginning at week 3 after injection. This may supply the source for the formation of osteoclasts. In our study, expression of vascular-related factors demonstrated the importance of MPS and LPS on inducing necrosis of the femoral head. Cartilage is an important structural component of the body which does not have blood vessels and nerves Sophia Fox et al., 2009. VEGFR-2 is expressed during articular cartilage growth and becomes quiescent at maturity. During osteoarthritis, the VEGFR-2 expression is also induced Lingaraj et al., 2010. A study showed that VEGFR-2 was also expressed in osteoblasts, osteoclasts and chondrocytes during the endochondral bone formation Maes and Carmeliet, 2008. During the process, hypertrophic chondrocytes die through induction of apoptosis and are replaced by osteoblasts Shapiro et al., 2005. Another study has shown that VEGF and its receptor play an important role in the initial stages of femoral head necrosis. Moreover, expression of CD31 (PECAM), a marker on endothelial cells of the major organs and large vessels, were shown to be increased- a sign of invasion of vascular cells into cartilage Melinte et al., 2012Pusztaszeri et al., 2006. In our study, the increased expression of vascular-related factors in the drug-treated mice group is an indication of invasion of blood vessel structures into the cartilage matrix, resulting in a changed cartilage structure.
Conclusion
This study shows that MPS, combined with LPS, can be used in mice to induce a model of early AVN of femoral head. We are aware that the mouse model does not show standardized pathogenesis of AVN of the femoral head. Nevertheless, our findings contribute to a better understanding about the process of AVN. This basic model should be suitable for investigating effective therapeutic modalities for AVN.
References
-
Y.
Azuma,
K.
Kaji,
R.
Katogi,
S.
Takeshita,
A.
Kudo.
Tumor necrosis factor-alpha induces differentiation of and bone resorption by osteoclasts. The Journal of biological chemistry.
2000;
275
:
4858-4864
.
-
B.
Beutler,
E.T.
Rietschel.
Innate immune sensing and its roots: the story of endotoxin. Nature Reviews Immunology.
2003;
3
:
169-176
.
-
K.
Bobacz,
I.G.
Sunk,
J.G.
Hofstaetter,
L.
Amoyo,
C.D.
Toma,
S.
Akira,
T.
Weichhart,
M.
Saemann,
J.S.
Smolen.
Toll-like receptors and chondrocytes: the lipopolysaccharide-induced decrease in cartilage matrix synthesis is dependent on the presence of toll-like receptor 4 and antagonized by bone morphogenetic protein 7. Arthritis and rheumatism.
2007;
56
:
1880-1893
.
-
C.T.
Chen,
N.
Burton-Wurster,
C.
Borden,
K.
Hueffer,
S.E.
Bloom,
G.
Lust.
Chondrocyte necrosis and apoptosis in impact damaged articular cartilage. Journal of orthopaedic research: official publication of the Orthopaedic Research Society.
2001;
19
:
703-711
.
-
JD
Esko,
K
Kimata,
L.
U..
Proteoglycans and Sulfated Glycosaminoglycans. In: Varki A, Cummings RD, Esko JD, et al., editors. Essentials of Glycobiology. Cold Spring Harbor (NY): Cold Spring Harbor Laboratory Press, Chapter 16.
2009
.
-
Q.
Espinassous,
E.
Garcia-de-Paco,
I.
Garcia-Verdugo,
M.
Synguelakis,
S.
von Aulock,
J.M.
Sallenave,
A.N.
McKenzie,
J.
Kanellopoulos.
IL-33 enhances lipopolysaccharide-induced inflammatory cytokine production from mouse macrophages by regulating lipopolysaccharide receptor complex. J.
2009;
Immunol183
:
1446-1455
.
-
T.
Hirayama,
L.
Danks,
A.
Sabokbar,
N.A.
Athanasou.
Osteoclast formation and activity in the pathogenesis of osteoporosis in rheumatoid arthritis. Rheumatology (Oxford).
2002;
41
:
1232-1239
.
-
T.
Ichiseki,
T.
Matsumoto,
M.
Nishino,
A.
Kaneuji,
S.
Katsuda.
Oxidative stress and vascular permeability in steroid-induced osteonecrosis model. J Orthop Sci.
2004a;
9
:
509-515
.
-
T.
Ichiseki,
T.
Matsumoto,
M.
Nishino,
A.
Kaneuji,
S.
Katsuda.
Oxidative stress and vascular permeability in steroid-induced osteonecrosis model. Journal of Orthopaedic Science.
2004b;
9
:
509-515
.
-
K.
Itoh,
N.
Udagawa,
K.
Kobayashi,
K.
Suda,
X.
Li,
M.
Takami,
N.
Okahashi,
T.
Nishihara,
N.
Takahashi.
Lipopolysaccharide promotes the survival of osteoclasts via Toll-like receptor 4, but cytokine production of osteoclasts in response to lipopolysaccharide is different from that of macrophages. J.
2003;
Immunol170
:
3688-3695
.
-
A.B.
J. Anthony Parker,
S. S. Tumeh.
AVASCULAR NECROSIS (Harvard Univeristy). 1988
.
-
M.A.
Kerachian,
D.
Cournoyer,
E.J.
Harvey,
T.Y.
Chow,
L.R.
Begin,
A.
Nahal,
C.
Seguin.
New insights into the pathogenesis of glucocorticoid-induced avascular necrosis: microarray analysis of gene expression in a rat model. Arthritis Res Ther.
2010a;
12
:
R124
.
-
M.A.
Kerachian,
D.
Cournoyer,
E.J.
Harvey,
T.Y.
Chow,
L.R.
Bégin,
A.
Nahal,
C.
Séguin.
Research article New insights into the pathogenesis of glucocorticoid-induced avascular necrosis: micro-array analysis of gene expression in a rat model. 2010b
.
-
H.K.
Kim,
H.
Bian,
J.
Aya-ay,
A.
Garces,
E.F.
Morgan,
S.R.
Gilbert.
Hypoxia and HIF-1α expression in the epiphyseal cartilage following ischemic injury to the immature femoral head. Bone.
2009;
45
:
280-288
.
-
Y.
Kobayashi,
K.
Maeda,
N.
Takahashi.
Roles of Wnt signaling in bone formation and resorption. Japanese Dental Science Review.
2008;
44
:
76-82
.
-
H.
Larjava,
V.J.
Uitto,
E.
Eerola,
M.
Haapasalo.
Inhibition of gingival fibroblast growth by Bacteroides gingivalis. Infect Immun.
1987;
55
:
201-205
.
-
K.
Lingaraj,
C.K.
Poh,
W.
Wang.
Vascular endothelial growth factor (VEGF) is expressed during articular cartilage growth and re-expressed in osteoarthritis. Annals of the Academy of.
2010;
Medicine
:
Singapore 39, 399-403
.
-
M.
Lotz,
S.
Hashimoto,
K.
Kuhn.
Mechanisms of chondrocyte apoptosis. Osteoarthritis and cartilage /.
1999;
OARS
:
Osteoarthritis Research Society 7, 389-391
.
-
B.T.
MacDonald,
K.
Tamai,
X.
He.
Wnt/β-catenin signaling: components, mechanisms, and diseases. Developmental cell.
2009;
17
:
9-26
.
-
C.
Maes,
G.
Carmeliet.
Vascular and Nonvascular Roles of VEGF in Bone Development.. In VEGF in Development (Springer New York).
2008;
:
79-90
.
-
R.
McManus.
Mechanisms of steroid action and resistance in inflammation and disease. The Journal of endocrinology.
2003;
178
:
1-4
.
-
R.
Melinte,
I.
Jung,
L.
Georgescu,
S.
Gurzu.
VEGF and CD31 expression in arthritic synovium and cartilage of human knee joints. Romanian journal of morphology and embryology = Revue roumaine de morphologie et embryologie.
2012;
53
:
911-915
.
-
S.J.
Millar,
E.G.
Goldstein,
M.J.
Levine,
E.
Hausmann.
Modulation of bone metabolism by two chemically distinct lipopolysaccharide fractions from Bacteroides gingivalis. Infect Immun.
1986;
51
:
302-306
.
-
K.
Miyanishi,
T.
Yamamoto,
T.
Irisa,
G.
Motomura,
S.
Jingushi,
K.
Sueishi,
Y.
Iwamoto.
Effects of different corticosteroids on the development of osteonecrosis in rabbits. Rheumatology.
2005;
44
:
332-336
.
-
F.
Nakazawa,
H.
Matsuno,
K.
Yudoh,
Y.
Watanabe,
R.
Katayama,
T.
Kimura.
Corticosteroid treatment induces chondrocyte apoptosis in an experimental arthritis model and in chondrocyte cultures. Clinical and experimental rheumatology.
2002;
20
:
773-781
.
-
M.P.
Pusztaszeri,
W.
Seelentag,
F.T.
Bosman.
Immunohistochemical expression of endothelial markers CD31, CD34, von Wille-brand factor, and Fli-1 in normal human tissues. The journal of histochemistry and cytochemistry : official journal of the Histochemistry Society.
2006;
54
:
385-395
.
-
W.
Qian,
M.
Li,
C.
Yan-Ping,
Y.
Lin,
L.
Wei,
W.
Xiao-Ning.
A rabbit model of hormone-induced early avascular necrosis of the femoral head. Biomedical and Environmental Sciences.
2008;
21
:
398-403
.
-
L.
Qin,
G.
Zhang,
H.
Sheng,
K.
Yeung,
H.
Yeung,
C.
Chan,
W.
Cheung,
J.
Griffith,
K.
Chiu,
K.
Leung.
Multiple bioimaging modalities in evaluation of an experimental osteonecrosis induced by a combination of lipopolysaccharide and methylprednisolone. Bone.
2006;
39
:
863-871
.
-
G.D.
Roodman.
Role of cytokines in the regulation of bone resorption. Calcified tissue international 53 Suppl.
1993;
1
:
S94-98
.
-
S.Y.
Ryoo,
S.H.
Jo,
A.
Kwak,
J.H.
Lee,
H.J.
Jhun,
A.S.
Sobti,
K.J.
Oh.
Effect of lipopolysaccharide (LPS) on mouse model of steroid-induced avascular necrosis in the femoral head (ANFH). Journal of microbiology and biotechnology.
2014;
24
:
394-400
.
-
K.
Sato,
H.
Takayanagi.
Osteoclasts, rheumatoid arthritis, and osteoimmunology. Current opinion in rheumatology.
2006;
18
:
419-426
.
-
M.
Shaarawy,
S.
Zaki,
M.
Sheiba,
A.M.
El-Minawi.
Circulating levels of osteoclast activating cytokines, interleukin-11 and transforming growth factor-beta2, as valuable biomarkers for the assessment of bone turnover in postmenopausal osteoporosis. Clinical laboratory.
2003;
49
:
625-636
.
-
I.M.
Shapiro,
C.S.
Adams,
T.
Freeman,
V.
Srinivas.
Fate of the hypertrophic chondrocyte: microenvironmental perspectives on apoptosis and survival in the epiphyseal growth plate. Birth defects research Part.
2005;
C
:
Embryo today: reviews 75, 330-339
.
-
A.J.
Sophia Fox,
A.
Bedi,
S.A.
Rodeo.
The Basic Science of Articular Cartilage: Structure, Composition, and Function. Sports Health.
2009;
1
:
461-468
.
-
N.M.
Stamatos,
I.
Carubelli,
D.
van de Vlekkert,
E.J.
Bonten,
N.
Papini,
C.
Feng,
B.
Venerando,
A.
d'Azzo,
A.S.
Cross,
L.X.
Wang.
LPS-induced cytokine production in human dendritic cells is regulated by sialidase activity. Journal of leukocyte biology.
2010;
88
:
1227-1239
.
-
R.
Todhunter,
S.
Fubini,
J.
Wootton,
G.
Lust.
Effect of methylprednisolone acetate on proteoglycan and collagen metabolism of articular cartilage explants. The Journal of rheumatology.
1996;
23
:
1207-1213
.
-
N.
Udagawa,
N.
Takahashi,
T.
Akatsu,
H.
Tanaka,
T.
Sasaki,
T.
Nishihara,
T.
Koga,
T.J.
Martin,
T.
Suda.
Origin of osteoclasts: mature monocytes and macrophages are capable of differentiating into osteoclasts under a suitable microenvironment prepared by bone marrow-derived stromal cells. Proceedings of the National Academy of Sciences of the United States of America.
1990;
87
:
7260-7264
.
-
R.J.
Hof,
S.H.
Ralston.
Cytokine-induced nitric oxide inhibits bone resorption by inducing apoptosis of osteoclast progenitors and suppressing osteoclast activity. Journal of bone and mineral research : the official journal of the American Society for Bone and Mineral Research.
1997;
12
:
1797-1804
.
-
S.
Viguet-Carrin,
P.
Garnero,
P.D.
Delmas.
The role of collagen in bone strength. Osteoporosis international : a journal established as result of cooperation between the European Foundation for Osteoporosis and the National Osteoporosis Foundation of the USA.
2006;
17
:
319-336
.
-
G.M.
Walsh.
Eosinophil apoptosis: mechanisms and clinical relevance in asthmatic and allergic inflammation. British journal of haematology.
2000;
111
:
61-67
.
-
J.J.
Wu,
D.R.
Eyre.
Structural analysis of cross-linking domains in cartilage type XI collagen. Insights on polymeric assembly. The Journal of biological chemistry.
1995;
270
:
18865-18870
.
-
X.
Wu,
M.A.
McKenna,
X.
Feng,
T.R.
Nagy,
J.M.
McDonald.
Osteoclast apoptosis: the role of Fas in vivo and in vitro. Endocrinology.
2003;
144
:
5545-5555
.
-
X.
Wu,
S.
Yang,
D.
Duan,
Y.
Zhang,
J.
Wang.
Experimental osteonecrosis induced by a combination of low-dose lipopolysaccharide and high-dose methylprednisolone in rabbits. Joint Bone Spine.
2008;
75
:
573-578
.
-
L.
Yin,
Y.-b.
Li,
Y.-s.
Wang.
Dexamethasone-induced adipogenesis in primary marrow stromal cell cultures: mechanism of steroid-induced osteonecrosis. CHINESE MEDICAL JOURNAL-BEIJING-ENGLISH.
2006;
EDITION-119
:
581
.
-
C.
Zalavras,
S.
Shah,
M.J.
Birnbaum,
B.
Frenkel.
Role of apoptosis in glucocorticoid-induced osteoporosis and osteonecrosis. Crit Rev Eukaryot Gene Expr.
2003a;
13
:
221-235
.
-
C.
Zalavras,
S.
Shah,
M.J.
Birnbaum,
B.
Frenkel.
Role of apoptosis in glucocorticoid-induced osteoporosis and osteonecrosis. Critical Reviews™ in Eukaryotic Gene Expression.
2003b;
13
.
-
J.
Zupan,
M.
Jeras,
J.
Marc.
Osteoimmunology and the influence of pro-inflammatory cytokines on osteoclasts. Biochem Med (Zagreb).
2013;
23
:
43-63
.
Comments
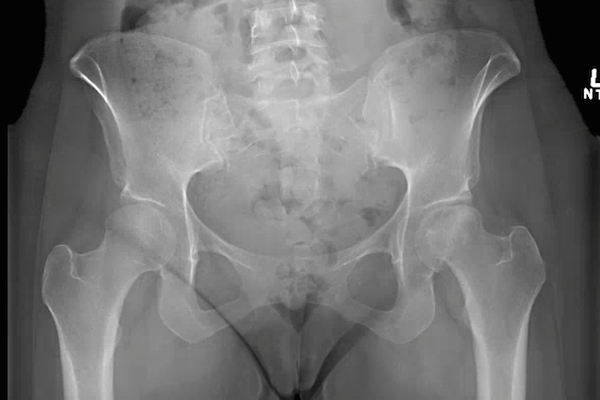
Downloads
Article Details
Volume & Issue : Vol 3 No 03 (2016)
Page No.: 548-556
Published on: 2016-03-25
Citations
Copyrights & License

This work is licensed under a Creative Commons Attribution 4.0 International License.
Search Panel
Pubmed
Google Scholar
Pubmed
Google Scholar
Pubmed
Google Scholar
Pubmed
Google Scholar
Pubmed
Google Scholar
Pubmed
Search for this article in:
Google Scholar
Researchgate
- HTML viewed - 5869 times
- Download PDF downloaded - 1719 times
- View Article downloaded - 6 times