In vitro and in vivo biocompatibility of Ti-6Al-4V titanium alloy and UHMWPE polymer for total hip replacement
Abstract
Introductions: Joint replacements have considerably improved the quality of life of patients with damaged joints. Over the past 30 years, there has been much effort and investigations in ways to repair damages in joints, including knee and hip joints. Materials for joint production have also been developed. Many improvements have been made in the joint replacement materials to increase their biocompatibility and longevity. This study is aimed at evaluating the in vitro and in vivo biocompatibility of Ti-6Al-4V titanium alloy and UHMWPE polymer used in total hip replacements.
Methods: Ti-6Al-4V titanium alloy and UHMWPE polymer were carefully washed with sterile distilled water then autoclaved. The materials were used directly or indirectly to evaluate pyrogens, endotoxins, animal cell cytotoxicity, gene mutation, animal cell transformation, DNA synthesis, immunogenicity, histology reactions, and immune response. All assays were performed according to ISO10993 guidelines.
Results: The results showed that Ti-6Al-4V titanium alloy and Chirulen 1020 UHMWPE polymer satisfied all criteria for implantable materials.
Introduction
Biomaterials are artificial or natural materials that can be used to produce implants which replace damaged or diseased biological structure. These biomaterials help improve the longevity and quality of life for a patient. In recent years, the biomaterials field has shown rapid growth with an increased demand, linked to unfortunate fractured bones and aging of patients. The number of implants, therefore, used for hip and knee replacements have increased greatly. In fact, 90% of the human population over the age of 40 suffers from degenerative diseases. Aged people often suffer tremendously and data show they have had an approximate 7-fold increase in the number of implants since 2002 (4.9 million in 2002 compared to 39.7 million in 2010) Aherwar et al., 2015.
At the present time, biomaterials can help solve the growing need for implants, since surgical implantation of biomaterials of appropriate shape and size can help restore the function of functionally compromised structures. However, the success of surgical implantation of joint or bone replacements relies on the skills of the orthopedic surgeon, not only to perform the surgery but also to select the best suitable replacement for the patient. Furthermore, the selection of an appropriate biomaterial for the fabrication of implants is precarious for the effectiveness and success of the implant. Almost all biomaterials used in the fabrication of different parts of the human body are fabricated from metals, polymers, ceramics and composites Black and Hastings, 2013Burg et al., 2000Jarcho, 1986Stenzel et al., 1974. However, both titanium alloys and UHMWPE polymers are the most popular materials for these applications.
Titanium alloys are lighter in weight than other metals and have good mechano-chemical properties. Titanium alloys have excellent biocompatibility and currently are the most widely used metals International and Davis, 2003. Titanium alloys can tightly integrate into bone and other tissues. Moreover, the rough surfaces of titanium alloy result in good osseointegration between the bone and the implant, when compared to smooth-surfaced implants, thus resulting in good clinical outcome after implant Ball et al., 2008. Ultra high molecular weight polyethylene (UHMWPE) is a useful thermoplastic polymer for biomaterial applications; it has high biocompatibility, chemical stability, impact strength and wear resistance, but low friction Onate et al., 2001.
This study aims to evaluate the biocompatibility of Ti- 6Al-4V titanium alloy and UHMWPE polymer, according to ISO 10993.
Materials and methods
Materials
Ti-6Al-4V titanium alloy (Grade 5) and Chirulen 1020 UHMWPE polymer were cut to small pieces, according to the ISO 10993-12. The materials were washed twice with double distilled water (ddH20), soaked in ddH20 for 24 h, then washed again in ddH20. They were then dried and autoclaved at 121°C for 20 mins. The sterile materials were directly used for further experiments or for producing material extracts.
Animals
Animals (rabbits and mice) were prepared according to the ISO10993. All animal manipulations were approved by the local ethics committee for animal care and use. Regarding the sex of the animals, for some short-term evaluations (less than 24hr), only female animals were used; for long-term evaluations (more than 24hrs), both males and females were used. There were 200 adult mice (30-35 g/mouse) and 20 adult rabbits (1.5-3.0 kg/rabbit) used for the total experiments in the study.
Biocompatibility test
Biocompatability of the materials was evaluated by an acute systemic toxicity test, intracutaneous reactivity test, subcutaneous implantation test and cytotoxicity test, according to the ISO 10993. Rectangular specimens (10 × 10 mm2) were used for extract liquid tests and bar type specimens for implantation tests.
Intracutaneous reactivity test
This test was done according to procedures per by the ISO 10993-10 (Annex B). Two healthy young adult albino rabbits, weighing not less than 2 kg, were used for each composition of titanium alloy specimens. The extract liquid was prepared by putting the specimen in a polar solvent (physiological saline). The extraction ratio of specimen to polar solvent was 4g/20 mL. The extraction condition was 37°C for 3 d; 0.2 mL of extract obtained with the polar solvent was injected intracutaneously at five sites on one side of each rabbit. Similarly, 0.2 mL of the polar solvent (control) was injected at five posterior sites on the same side of each rabbit. The appearance of each injection site was observed immediately after injection and at 24, 48 and 72 h after injection.
Implantation in subcutaneous tissue test (Histological evaluation)
Implantation in subcutaneous tissue was evaluated using procedures indicated by the ISO 10993-6. Three SDrats weighing approximately 160 - 230 g were used in this study. The implantations were performed according to the procedure and principles provided by ISO 10993-6. The animals were cared for in compliance with an approved protocol from the Institutional Animal Care Committee. Prior to surgery, the animals were anesthetized, and then the surgical site was shaved and cleaned with iodine. Local anesthesia using 2% lidocaine was administered to the tibial metaphysis where the implants were placed. An incision of the skin was done and subcutaneous pockets were made by blunt dissection. One bar-shaped sample were inserted in each rat’s subcutaneous tissue. The rats were sacrificed at 12 weeks after implant surgery. Each sample-tissue section was removed from the rat and fixed with 10% formalin for 24 h. After removing the implanted material, the section was stained with hematoxylin& eosin and histomorphological analysis was done.
Cell culture
The 3T3, CHO-K1 cell lines were obtained from the American Type Culture Collection (ATCC). Cells were cultured separately in Dulbecco’s modified Eagle’s Medium (DMEM) and MEM medium (Sigma-Aldrich) containing 10% fetal bovine serum (FBS; Sigma- Aldrich), 1% penicillin (10,000 units)/streptomycin (10 mg/mL; Sigma-Aldrich) and 1% 200 mM L-glutamine (Sigma-Aldrich), and maintained in humidified atmosphere containing 5% CO2 at 37°C. The culture medium was renewed twice per week. When cells reached confluence, a diluted Trypsin-EDTA (Ethylene Diamine Tetra Acetic acid; 0.5 g/L Trypsin and 0.2 g/L EDTA; Sigma-Aldrich) was used to detach cells from the bottom of the culture flasks and 1/5 of the total cells were transferred into a new tissue culture flask.
Pyrogenicity and endotoxin
Pyrogenicity were assessed as material-mediated pyrogenicity using traditional biocompatibility extraction methods (e.g. 50°C for 72 h; 70°C for 24 h, or 121°C for 1 h per ISO 10993-12), using a pyrogenicity test such as the one outlined in the USP 34 <151> Rabbit Pyrogen Test or an equivalent validated method. Briefly, 8 rabbits were used for pyrogenicity testing for titanium alloy and 8 rabbits for polymer. The materials were put under the rabbit skin. Body temperature was measured at the rabbit’s anus; body temperature was measured for 3 months at 1 week before material graft, and weekly after material transplantation. For endotoxin testing, the materials were evaluated via LAL assay. Briefly, the materials were soaked in DMEM/F12 medium at 37°C for 3 d. The supernatant was used to evaluate endotoxin by LAL assay.
Cytotoxicity assay
Cytotoxicity test was performed by the procedures indicated by the ISO 10993-5. Extraction media of materials were prepared with the serum free DMEM/F12 media at the ratio of 4g/20mL (or 6cm/mL) at 370 C and for 3 d. Sodium Dodecyl Sulphate (100μg/ml) was used as a positive control. A minimum of three replicates were used for test samples and controls. All procedures were performed under aseptic conditions. 3T3 cell line (from ATCC, US) was used for this assay. When the cells proliferated up to 80% confluence, the supernatant culture medium was discarded and the cells were incubated with extracted medium for 24 h. Determination of cytotoxicity was performed via neutral red assay; cells incubated with supernatant for 24 h were stained with neutral red dye and samples were measured at 540 nm in the biophotometer (DTX880, Beckman Coulter, US).
Genotoxicity and carcinogenicity
The materials were soaked in DMEM/F12 medium at 37°C for 3 d and supernatant was used for the assay. The genotoxicity and carcinogenicity were evaluated by Ames/Salmonella assay, according to ISO 10993-3 (OECD471).
Carcinogenicity was evaluated in animal cells (CHO-K1). The supernatant was diluted in various concentrations, including 100%, 50%, 25%, 12.5% and 6.25% of supernatant in cell culture medium. Regular cell culture was used as a negative control and Ethyl methane sulfonate (EMS, 0.2g/ml) was used as a positive control. The assay was performed according to the OECD 476.
Genome toxicity and in vitro mutation
Genome toxicity and in vitro mutation were assessed by transformation of animal cells and DNA synthesis in murine liver cells. All mice used in this assay were controlled for body weight; during the investigation period the body weight did not exceed 20% of body weight from the initial time point. There were 3 groups of mice, each with 12 mice. The groups were: group I- negative control, group II- titan alloy supernatant, and group III- polymer supernatant. Mice were subcutaneously implanted with the above samples and monitored for 90 d. The liver tissues were collected at day 30 and day 90 after implant. In the in vitro mutation assay, Methylmethasulfonate (MMS) was used as a positive control. The liver cells were induced with 10 ug/mL MMS for 10 min at 37°C. The liver cells were isolated from liver tissue by pressing the liver tissue between two lanes. The cells were resuspended in 0.9% NaCl. The cell suspension was centrifuged at 800 rpm for 5 min and the cell pellet was then resuspended in 120 ul of 0.5% low melting agarose at 37°C. The cell solution was spread in the lane pre-coated with 1.5% agarose. This lane was put at 4°C for 5 min then soaked in lysing solution (2.5M NaCl, 100 mM EDTA, 10 mMTris-HCl, pH 10, 1% sodium sarcosinate with 1% Triton X-100 and 10% DMSO) for 1 h. Then, the lane was treated with alkaline solution (pH>13) for 20 min. This lane was used in electrophoresis at 0.7 V/cm, 300 mA for 20 min, and neutralized with 0.4M Tris-HCL (pH 7.5) and fixed in 100% alcohol. Finally, DNA was stained with ethidium bromide (50ug/ml) and imaged by UVP Gel imaging.
Immunogenicity of materials
The immunogenicity of materials were evaluated according to the ISO 10993-20 (2006). The evaluation was performed for acute immune response. There were 15 mice used in this assay. Materials were subcutaneously implanted with materials for 24 h. The spleen and thymus were collected to evaluate their weight, compared to control animals.
Immune response of mouse models for materials
The subchronicimmunotoxicity evaluation was based on guidelines in the ISO 10993-11:2006 and ISO 10993-20: 2006. Fourty mice were divided into four groups of two materials and six mice in control groups (three mice per sex). Animals were anesthetized with Ketamine (25 mg/kg dose) and implanted the materials subcutaneously. After twenty-one days, serums of mice were collected and used in immunoprecipitation assay with material extracts.
Statistical analysis
All collected data were analyzed with Excel 2000. The difference between control and experimental groups was assessed using a student t-test (α = 0.05).
Results and Discussions
Effects of materials on fever in rabbits
Rabbits are animals that are sensitive to changes in environmental conditions and their bodies respond very quickly especially during fevers. The normal temperature of a rabbit varies from 38.5°C to 39.7°C, depending on the temperature of the environment. At 2 d after material implantation, fever response in both control and experimental groups (with titanium and polymer implantation) did not exceed temperature thresholds (i.e. over 3.3°C after implantation compared to before).
At 2 weeks after implantation, initially there was no rabbit with fever, swelling or shock. To confirm these results, all rabbits were continuously followed up to 12 weeks. There was no change as well (e.g. no signs related to fever). The implanted rabbits showed normal growth. At 12 weeks post implantation, the body temperatures of the implanted rabbits were not significantly different than control rabbits, and not significantly different to temperatures in pre-grafted animals. These results showed that both titanium and polymer materials did not cause any acute immune reactions from 1 to 15 days post implant, nor did they cause fever after 12 weeks.
Histological reaction of materials when subcutaneously grafted
After 12 weeks of transplantation of materials under the skin, the local tissues at the grafted sites were collected and analyzed. For intramuscular implantation, as depicted in Figure 1 , the cells in the tissues were not necrotic, shrunk or swollen, suggesting that the membranes and nuclei were intact. Inflamed cells were not detected in these tissues ( Figure 1 ). Moreover, small broken species of grafted materials were not found at these sites. The structure of the muscular tissues were also unchanged at the grafted sites. These results were similar for both titanium and polymer materials.
The subcutaneous implantation also showed that both titanium and polymer had similar results. All skin samples obtained at grafted sites showed undamaged skin structure. Moreover, there were no immune cells present at these sites. To assess collagen synthesis at grafted skin sites, the tissues were collected and stained with Trichrome staining. The results showed that collagen synthesis at these sites was not significantly different to non-grafted sites of skin ( Figure 2 ).
Endotoxin testing
The results showed that both polymer and titanium materials did not form gel like negative control, contrast to the positive control sample in the LAL assay ( Figure 3 ). As guideline of LAL assay, both materials’ endotoxins were lower than 0.25EU/ml.
In vitro cytotoxicity
The results showed that 3T3 cell morphology did not change after 24 h of culture with extracts of both polymer and titanium, compared to normal culture (without extract supplement). 3T3 cells adhered to and spread normally on the surface of flasks, and maintained a homogeneous population. Cytoplasm was visible by light microscopy. There were no dead cells observed in suspension. However, in the positive control (cells treated with SDS) the cells and cytoplasmic membrane showed necrosis ( Figure 4 ).
The neutral red assay showed that the material extracts affected 3T3 cell viability. The left and the right mean of the blanks were 4.04% for polymer and 10.893% for titanium (<15%). The mean OD540 of the blanks were 0.309 (≥ 0.3) for polymer and 0.309 (≥ 0.3) for titanium. Cell viability at the highest concentration of extract (100% extract) and the lowest concentration for both polymer and titanium extract were higher than 70% of the control group ( Figure 5 ). In constrast, SDS caused cell death in up to 100% of cells. These results showed that the materials were not cytotoxic.
Genotoxicity
The genotoxicity of materials was evaluated by Ames assay. The results showed that in the treatment groups (titanium or polymer extracts, with or without S9 extraction), the index of bacteria reverse mutation was less than one ( Table 1 ). The results were significantly different compared to positive and negative controls. In the positive control group, the index of bacteria reverse mutation was 15.19 (without S9) and 17.39 (with S9) ( Figure 6 ). The results showed that both titanium and polymer, with or without S9 extraction supplement, did not cause mutations in bacteria.
In vitro mammalian cell gene mutation
Results showed that colony formation of CHO-K1 cells in the treatment group (titanium or polymer) was less than 90. It was significant different from the positive and negative control groups. It was recorded that quantity of colonies from 1 million cells in positive groups (supplied MMS) was more than titanium/polymer treated groups. MMS caused mutations of CHO-K1 cells, from a TK+/- genotype into a TK-/- genotype. The mutation of thymidine kinase of CHO-K1, induced by MMS, resulted in a higher survival rate of cells in pyrimidine analogue trifluorothymidine (TFT) medium. On the contrary, titanium and polymer extracts did not cause mutations of thymidine kinase in CHO-K1 cells. In addition, the mutation frequency of titanium and polymer treatment groups was less than 1, which was significantly different from the positive control group ( Figure 7 , Table 2 ). The results indicated that titanium and polymer extracts did not induce gene mutations in the cultured mammalian cells.
DNA damage evaluation by comet assay
After 90 d of induction, DNA damage was observed in the positive control group, with distinguishable images of head and tail. The positive control (MMS) caused double and single stranded DNA damage in cell nuclei. Regarding titanium and polymer groups, comet images were not observed ( Figure 8 ). Table 3 shows DNA damage of mouse liver cells by tail moment. The results show that titanium and polymer did not cause DNA damage or mutations in mouse liver cells after 90 d.
Immunogenicity
The results show that spleen weight of mice in titanium and polymer groups were not significantly different from those in the control group (0.1429 ± 0.0463 g, 0.1019 ± 0.0182 g and 0.1279 ± 0.0247 g, respectively; p > 0.05). Likewise, the thymus weights in the experimental groups was not significantly different from controls (0.0485 ± 0.0027 g, 0.0516 ± 0.0150 g, and 0.0526 ± 0.0044 g, respectively; p > 0.05) ( Figure 9 ). Thus, titanium alloy and polymer do not affect mouse spleen and thymus weight after 24-h implantation. These results show that Ti-6Al-4V titanium alloy and UHMWPE polymer do not induce immunogenicity in mice.
Immune response to materials in mice
At 21-d post implantation, material cubes and serum were collected from mice. Mouse serum and material leach were put into wells in the same agarose gel. The gel plates were kept from light for 24h in an incubator.
After 24 h, there was no visible antibody-antigen complex in all plates of the three groups (titanium, polymer and control). Thus, results showed that mice from implanted and control groups did not produce antibody to materials grafted in their bodies.
Discussion
Materials such as titanium and polymer composites are among the most popular ones used in medical devices. Recently, several medical devices have been widely produced for replacement of knee and hip joints, and there has been development of new tools used in orthopedics. Titanium alloy (Ti-6Al-4V) and Polymer (UHMWPE) have been produced and supplied worldwide as materials for medical devices in humans for joint replacements. However, local conditions and facilities that produce these materials may affect their quality, especially biocompatibility. Therefore, this study was aimed to re-evaluating the biocompatibility of these two materials before use in further experimentations or processing.
As materials for medical devices, both titanium and polymer were evaluated according to the ISO 10993. Several assays were used to evaluate the materials, including assessment of fever in rabbits, endotoxin, histological reactions, in vitro cytotoxicity, genotoxici- ty, in vitro mammalian cell gene mutation, DNA damage, immunogenicity, and long term degradation. All the assays showed that both titanium and polymer satisfied the criteria for materials used in medical devices.
First, titanium and polymer were implanted under the skin of a rabbit to test fever- causing capability. Both materials could not cause fever in rabbits at 12 weeks post implantation. These results are similar to previously published studies Chakraborty et al., 2011Stangl et al., 2004. Endotoxin testing is another essential test for materials used in medicine van Putten et al., 2011. Endotoxin is a complex lipopolysaccharide (LPS) found in the outer cell membrane of gramnegative bacteria. They are approximately 10 kDa in size, but readily form large aggregates up to 1000 kDa. Endotoxins have a high heat stability, making it impossible to destroy them under regular sterilizing conditions Gorbet and Sefton, 2005. The endotoxin level of titanium and polymer after local treatment was lower than 0.25EU/mL, which satisfies the criteria for medical devices. Thus, our sterile procedures and processing methods of the biomaterials rendered them safe and appropriate for use in medical applications.
Next, materials were evaluated for their effects on in vitro cytotoxicity. In this assay, 3T3 fibroblast cells were used to test cytotoxicity of extracts obtained from titanium and polymer. The results showed that both materials did not affect 3T3 cell morphology or growth. Although there are new suggested methods to assess cytotoxicity of materials Miller et al., 2015, the cytotoxicity assay based on 3T3 cell proliferation is still considered a traditional method, and entails measuring neutral red uptake Borenfreund et al., 1988Borenfreund and Puerner, 1985Repetto et al., 2008.
Although the effect of the material extracts on cell morphology and proliferation are important criteria for materials used in medicine, effects of the materials on the genome (e.g. genotoxicity, gene mutation and DNA damage) are also essential criteria. The greatest long-term risk of biomaterials is related to tumorigenesis. Effects of biomaterials on gene mutation and DNA damage are considered direct factors for tumorigenesis. Therefore, the genotoxicity of materials were evaluated for titanium alloy and polymer. Using Ames assay, comet assay and the in vitro mutation assay on CHO-K1 cell lines, we showed that both titanium alloy and polymer used in this study did not cause an increase of mutations in animal cells nor did they cause DNA damage. The Ames assay is a standard assay to evaluate long-term effects of mutagens; it is also used to assess mutagenicity of an agent before use in medicine Kettering and Torabinejad, 1995Kilbey et al., 2012McCann et al., 1975. Similarly, the comet assay is a standard assay to evaluate DNA damage and repair Collins, 2004Hellman et al.,1995Olive and Banáth, 2006. Our results were in concordance with previously published studies that indicate Ti-6Al-4V titanium to be safe- without cytotoxicity or genotoxicity Velasco-Ortega et al., 2010.
Besides cytotoxicity and genotoxicity, histological reactions induced by materials are necessary to consider. Histological reactions relate to the effects of materials to cause local changes in tissue structures at the grafted sites, and can be useful tests for new materials before use in medicine. In this study, titanium and polymer were implanted into tibia muscle and under the skin to evaluate histological reactions at these sites. The results showed that there were not any serious reactions recorded at both muscle and skin sites. There were no observed inflammatory cells, immune cells and abnormal cells, and no collagen synthesis recorded at the skin after 12 weeks of material implantation.
Lastly, we evaluated the immunogenicity of the materials, following subcutaneous implantation in mice. The immunogenicity of both materials were evaluated by changes in weight of both spleen and thymus. The results showed that material implantation did not cause the inflammation in mice. The results are confirmed by the observation that white blood cell total did not significantly change during the study, as well as compared to pre-implantation and compared to non-grafted mice. Other studies have also shown that titanium alloy Granchi et al., 2008Williams, 2001Xu et al., 2013 and polymer Dahmen et al., 1997Wiesli and Özcan, 2015 are non-immunogenic materials.
Conclusion
Biocompatible materials are essential for development of medical devices. In recent years human tissues, such as knee and hip replacements, have been artificially produced by biocompatible materials. Titanium alloy Ti-6Al-4V and Polymer UHMWPE are two popular materials for these applications. This study evaluated the biocompatibility of Ti-6Al-4V titanium alloy and UHMWPE polymer according to ISO 10993. Our results confirm that both titanium alloy Ti-6Al-4V and polymer UHMWPE are biocompatible materials and thus suitable for medical devices.
References
-
A.
Aherwar,
A.K.
Singh,
A.
Patnaik.
Current and future biocompatibility aspects of biomaterials for hip prosthesis. 2015
.
-
M.
Ball,
D.M.
Grant,
W.J.
Lo,
C.A.
Scotchford.
The effect of different surface morphology and roughness on osteoblast-like cells. J Biomed Mater Res A.
2008;
86
:
637-647
.
-
J.
Black,
G.
Hastings.
Handbook of biomaterial properties. Springer Science & Business Media.
2013
.
-
E.
Borenfreund,
H.
Babich,
N.
Martin-Alguacil.
Comparisons of two in vitro cytotoxicity assays-the neutral red (NR) and tetrazolium MTT tests. Toxicology in vitro.
1988;
2
:
1-6
.
-
E.
Borenfreund,
J.A.
Puerner.
Toxicity determined in vitro by morphological alterations and neutral red absorption. Toxicology letters.
1985;
24
:
119-124
.
-
K.J.
Burg,
S.
Porter,
J.F.
Kellam.
Biomaterial developments for bone tissue engineering. Biomaterials.
2000;
21
:
2347-2359
.
-
A.
Chakraborty,
B.
Kundu,
D.
Basu,
T.K.
Pal,
S.K.
Nandi.
In vivo bone response and interfacial properties of titanium-alloy implant with different designs in rabbit model with time. Indian J Dent Res.
2011;
22
:
277-284
.
-
A.R.
Collins.
The comet assay for DNA damage and repair. Molecular biotechnology.
2004;
26
:
249-261
.
-
K.
Dahmen,
N.
Maurin,
H.
Richter,
C.
Mittermayer.
Screening of biomedical polymer biocompatibility in NMRI-mice peritoneal cavity: A comparison between ultra-high-molecular-weight polyethylene (UHMW-PE) and polyethyleneterephthalate (PET). Journal of Materials Science: Materials in Medicine.
1997;
8
:
239-245
.
-
M.B.
Gorbet,
M.V.
Sefton.
Endotoxin: the uninvited guest. Biomaterials.
2005;
26
:
6811-6817
.
-
D.
Granchi,
E.
Cenni,
D.
Tigani,
G.
Trisolino,
N.
Baldini,
A.
Giunti.
Sensitivity to implant materials in patients with total knee arthroplasties. Biomaterials.
2008;
29
:
1494-1500
.
-
B.
Hellman,
H.
Vaghef,
B.
Boström.
The concepts of tail moment and tail inertia in the single cell gel electrophoresis assay. Mutation Research/DNA Repair.
1995;
336
:
123-131
.
-
A.
International,
J.R.
Davis.
Handbook of materials for medical devices. ASM international.
2003
.
-
M.
Jarcho.
Biomaterial aspects of calcium phosphates. Properties and applications. Dental Clinics of North America.
1986;
30
:
25-47
.
-
J.D.
Kettering,
M.
Torabinejad.
Investigation of mutagenicity of mineral trioxide aggregate and other commonly used root-end filling materials. Journal of endodontics.
1995;
21
:
537-539
.
-
B.J.
Kilbey,
M.
Legator,
W.
Nicholson,
C.
Ramel.
Handbook of mutagenicity test procedures (Elsevier). 2012
.
-
J.
McCann,
E.
Choi,
E.
Yamasaki,
B.N.
Ames.
Detection of carcinogens as mutagens in the Salmonella/microsome test: assay of 300 chemicals. Proceedings of the National Academy of Sciences.
1975;
72
:
5135-5139
.
-
F.
Miller,
U.
Hinze,
B.
Chichkov,
W.
Leibold,
T.
Lenarz,
G.
Paasche.
Validation of eGFP fluorescence intensity for testing in vitro cytotoxicity according to ISO 10993-5. J Biomed Mater Res B Appl Biomater.
2015
.
-
P.L.
Olive,
J.P.
Banáth.
The comet assay: a method to measure DNA damage in individual cells. NATURE PROTOCOLS-ELECTRONIC EDITION-.
2006;
1
:
23
.
-
J.
Onate,
M.
Comin,
I.
Braceras,
A.
Garcia,
J.
Viviente,
M.
Brizuela,
N.
Garagorri,
J.
Peris,
J.
Alava.
Wear reduction effect on ultra-high-molecular-weight polyethylene by application of hard coatings and ion implantation on cobalt chromium alloy, as measured in a knee wear simulation machine. Surface and Coatings.
2001;
Technology142
:
1056-1062
.
-
G.
Repetto,
A.
del Peso,
J.L.
Zurita.
Neutral red uptake assay for the estimation of cell viability/cytotoxicity. Nature protocols.
2008;
3
:
1125-1131
.
-
R.
Stangl,
A.
Pries,
B.
Loos,
M.
Muller,
R.G.
Erben.
Influence of pores created by laser superfinishing on osseointegration of titanium alloy implants. J Biomed Mater Res A.
2004;
69
:
444-453
.
-
K. H.
Stenzel,
T.
Miyata,
A. L.
Rubin.
Collagen as a biomaterial. Annual review of biophysics and bioengineering.
1974;
3
:
231-253
.
-
S.M.
Putten,
M.
Wubben,
J.A.
Plantinga,
W.E.
Hennink,
M.J.
van Luyn,
M.C.
Harmsen.
Endotoxin contamination delays the foreign body reaction. J Biomed Mater Res A.
2011;
98
:
527-534
.
-
E.
Velasco-Ortega,
A.
Jos,
A.M.
Camean,
J.
Pato-Mourelo,
J.J.
Segura-Egea.
In vitro evaluation of cytotoxicity and genotoxicity of a commercial titanium alloy for dental implantology. Mutat Res.
2010;
702
:
17-23
.
-
M.G.
Wiesli,
M.
Özcan.
High-Performance Polymers and Their Potential Application as Medical and Oral Implant Materials: A Review. Implant dentistry.
2015;
24
:
448-457
.
-
D.F.
Williams.
Titanium for medical applications. In Titanium in medicine (Springer).
2001;
:
13-24
.
-
J.
Xu,
X.-J.
Weng,
X.
Wang,
J.-Z.
Huang,
C.
Zhang,
H.
Muhammad,
X.
Ma,
Q.-D.
Liao.
Potential use of porous titanium- niobium alloy in orthopedic implants: preparation and experimental study of its biocompatibility in vitro. PloS one.
2013;
8
:
e79289
.
Comments
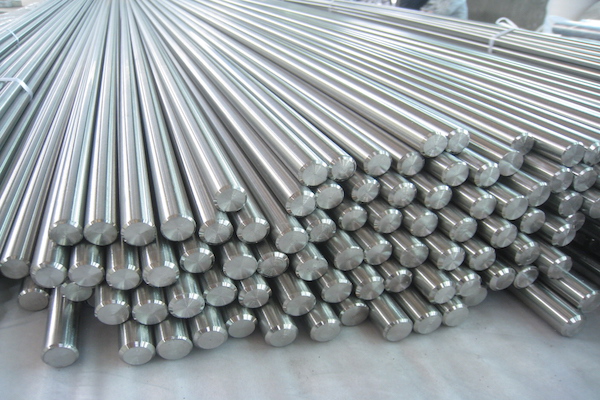
Downloads
Article Details
Volume & Issue : Vol 3 No 03 (2016)
Page No.: 567-577
Published on: 2016-03-25
Citations
Copyrights & License

This work is licensed under a Creative Commons Attribution 4.0 International License.
Search Panel
- HTML viewed - 11728 times
- Download PDF downloaded - 2318 times
- View Article downloaded - 6 times